The modern quest for Promethean fire is underway in an anonymous office park in Foothill Ranch, California, an hour southeast of Los Angeles. In the park, along a meandering drive, you will find a huge, modern warehouse building with “TAE Technologies” emblazoned on the door. Inside, you will find a 100-foot-long, $200 million fusion-energy experiment named Norman. And in a second-floor office, upstairs from that looming machine, you will find the would-be Prometheus himself: Michl Binderbauer, TAE’s boyish-looking and relentlessly upbeat co-founder and CEO.
In keeping with the rest of the TAE headquarters, his office looks spare, functional, and slightly unfinished. The most striking object adorning the room is a large whiteboard next to his desk, its upper three-quarters jammed with equations and crude engineering sketches for upgraded fusion devices. Across the bottom, within the low reach of little hands, the business notations give way to a whimsical set of drawings created by Binderbauer’s two young children. At first he seems bemused that these silly scrawls, of all things, are what draws my attention. Then he rolls with it and recalls a recent conversation with his 10-year-old son, who had just learned about climate change in school:
“He’s a precocious kid. He asked me, ‘Is this right? Is this really what’s happening?’ I told him, ‘I hope not. If Daddy can help, it may not happen.’ So now when people ask him what I do, he says, ‘My dad is trying to save the planet’.”
Like so much of what Binderbauer, 51, says, the story sounds too good to be true and yet utterly sincere. As he sees it, his save-the-planet strategy is no do-gooder’s dream; it is the perfectly logical end point of a perfectly rational analysis about the state of the world. His indisputable starting proposition is that the world needs a growing supply of energy. Then he asks, how are we going to generate it? Coal, oil, and natural gas are causing disruptive and deadly climate changes, so fossil fuels cannot be the future. Nuclear power produces no direct carbon emissions, but it leaves behind radioactive waste and—fairly or not—faces bitter public resistance.
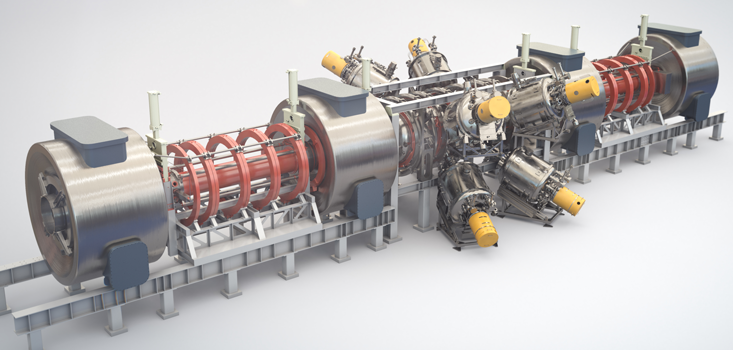
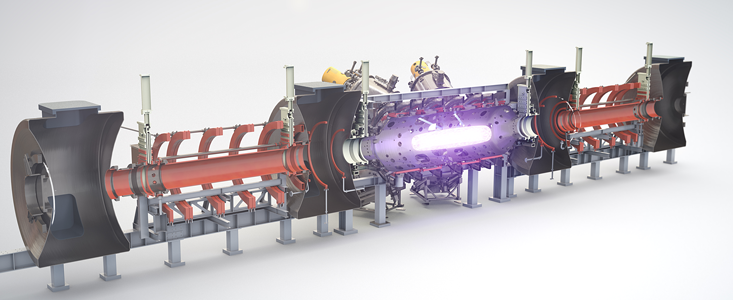
Solar and wind power are environmental darlings, but expanding them significantly will require vast new infrastructure to make their clean electricity available when and where it is needed. “Relying solely on solar and wind is a fool’s hope, I think,” Binderbauer says. Several recent studies, including a major technical analysis in the journal Proceedings of the National Academy of Sciences, back up his assessment: We simply do not know how to build an electric grid that can handle the inconsistent way that the sun shines and the wind blows. Even if renewables come to generate the majority of our electricity, they need to be combined with so-called dispatchable power that can be switched on at any time.
All those considerations, taken together, imply that we need something completely new in the global energy mix. Binderbauer argues that the most realistic something is fusion. Physicists know that smashing together atomic nuclei (the ultra-dense centers of atoms) and causing them to merge can release a tremendous amount of energy, far more than is possible by burning coal or natural gas, with no carbon pollution. They have dramatic proof that the process works: Fusion is what powers the sun, and it is what gives a hydrogen bomb an explosive kick. But after eight decades of research, nobody has been able to create a machine that can make fusion happen gently, steadily, and efficiently enough to generate electricity.
“I started to understand why the available concepts were flawed.”
Binderbauer aims to be the one to crack the code. He predicts that TAE could go from Norman to a demonstration fusion reactor within five years, and then—if everything goes right—to a prototype for a commercial fusion power plant another five years or so after that. His timetable is at least a decade faster than that of the biggest government-run fusion efforts. A handful of other private fusion startups have recently sprung up, promising their own speedy paths to fusion, but TAE has the most funding—more than $600 million—and, laid out here in front of me, the only fully operational test reactor. The company also has the most audacious plan, pursuing a novel type of fusion reaction that would take in hydrogen and boron (common elements of water and laundry detergent) and spit out nearly limitless clean energy.
Even within the far-out world of fusion energy, Binderbauer is operating on the fringe. He acknowledges that he could end up more like Icarus than Prometheus, flying too close to the sun and falling. Still, he sees no other option. “Our generation may go down in the history books as the ones who really ruined the planet on a massive scale,” he says. “Maybe I can’t change it, but hopefully I can at least come up in the same history book paragraph that adds, ‘… but there were some who really tried’.”
Restlessly reaching for better ideas seems to run in the Binderbauer family. Michl was born in Salzburg, Austria, but was constantly on the move through his childhood as his father kept relocating across Europe to explore new businesses. Eventually he took a bigger leap and settled for a year in Dallas, where American-style ambition took hold in 16-year-old Michl’s brain. That peripatetic upbringing left him with an unplaceable accent, a strange mashup that somehow resulted in something that sounds like a musical Danish lilt.
From Texas, Binderbauer embarked on his own roaming—to Southern Methodist University, the University of California at Irvine, and almost to graduate school in astrophysics at Johns Hopkins in 1990. He reversed his decision swiftly on his first visit to campus, when he was dismayed by a drive through nearby Baltimore neighborhoods full of homeless people and boarded-up buildings. He returned to Irvine, unsure of what to do next, when he had a transformative encounter with Norman Rostoker, his former physics professor.
Rostoker had been fascinated by the possibility of harnessing fusion energy since his early research days in the 1950s. Now he was looking for a Ph.D. student to finally help him put that idea into action. Binderbauer signed on, and got pulled into his mentor’s worldview. “About a year into it, I really started to appreciate the basic idea, what fusion could do for humanity,” he says. “But more important, I started to understand what wouldn’t work, and why the available concepts were flawed.”
Ever since then, Binderbauer’s life has been guided by those twin fixations: The world needs fusion, and the world also needs a better way to make it happen.
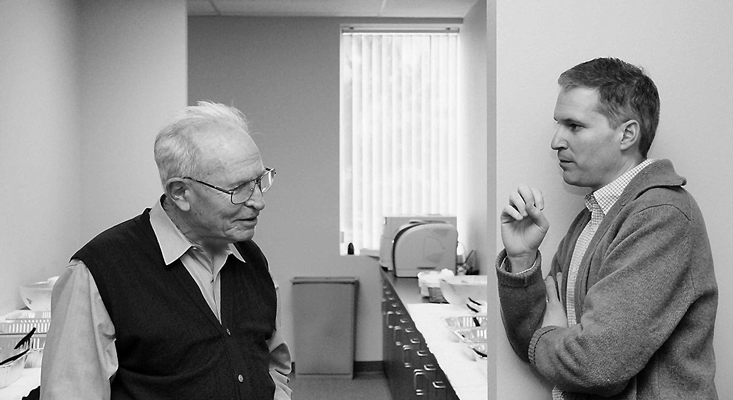
The need for fusion was clear enough even in 1990. Scientists had already accumulated abundant evidence that carbon dioxide from fossil fuels was disrupting Earth’s climate. At the same time, public sentiment in the United States had been turning sharply hostile to nuclear reactors since the Three Mile Island accident in 1979. The catastrophic explosion of the Soviet Chernobyl reactor in 1986 cemented a widespread attitude that nuclear energy is unsafe. There was also the hot-potato issue of where to put spent nuclear fuel, which remains highly radioactive. In 1987, the U.S. Congress designated Yucca Mountain in Nevada as the nation’s future nuclear waste repository. Nevada residents immediately protested and the state filed a lawsuit to block the plan. Three decades later, the site still has not opened.
In sharp contrast to nuclear energy, fusion looks downright benign. “It doesn’t have the nuclear footprint, it doesn’t have the waste stream, and it can’t have a meltdown or fallout,” Binderbauer notes. The problem is that fusion is drastically harder to achieve than the fission reactions that power a nuclear reactor—which is why nuclear power is a reality today, while fusion remains a promise for tomorrow.
Relying solely on solar and wind is a fool’s hope.
To get a nuclear reactor going, pretty much all you need is a stash of radioactive fuel. If you throw together a heap of purified uranium, it will start generating heat all by itself; the biggest challenge is preventing it from generating too much heat and melting down. To make fusion happen, on the other hand, you need an intense kickstart: You need to collide pairs of atomic nuclei so forcefully that they stick together, releasing some of their latent energy. The sun gets those collisions going with its enormous gravitational pressure. A hydrogen bomb does the job by whacking the nuclei with an atomic explosion.
Making fusion happen in a steady, controlled way here on Earth involves a seemingly contradictory combination of delicacy and brutality. It requires a special kind of reactor that can heat nuclei to millions of degrees while containing them so that they don’t fly apart before they have a chance to collide. In other words, you need to put a lot of energy into a fusion reactor before you can get any energy out. Many experiments have now succeeded in sparking fusion reactions, but none of them has managed to get more energy out than the amount that goes in, the critical tipping point known as breakeven.
Still, fusion researchers have made a great deal of progress in figuring out how to get there. In particular, they have zeroed in on the most promising nuclear reaction for achieving breakeven. The favored reaction uses two heavy versions of hydrogen, known as deuterium and tritium. The two of them both have positive charges, which normally causes them to repel each other. But if you heat them to temperatures around 100 million degrees Celsius, they will occasionally come so close together that the short-range attraction of the strong nuclear force overwhelms the long-range effects of repulsion, causing them to fuse.
Researchers zeroed in on deuterium-tritium fusion because it is one of the easiest reactions to trigger. When deuterium combines with tritium, they also release a lot of energy: One kilogram of deuterium contains the energy equivalent of 29 million kilograms of coal. Further adding to the appeal of this approach, both types of nuclei are easily obtainable. Deuterium can be extracted from ordinary water; tritium can be produced by irradiating lithium, a straightforward laboratory process.
Pretty much every major fusion experiment since the 1970s has been designed with deuterium-tritium fusion in mind. In the south of France, an international consortium is currently constructing a $25 billion behemoth called ITER (formerly the International Thermonuclear Experimental Reactor), which is on track to become the world’s largest fusion plant when completed in 2025. It will run on deuterium-tritium. Private startups like Commonwealth Fusion Systems in Cambridge, Massachusetts, and General Fusion in Burnaby, Canada, take different technological approaches to fusion, but they, too, are planning machines that will run on deuterium and tritium. The choice of fuel is the one thing that the entire fusion world can agree on.
Well, almost. The choice of fuel lies at the heart of Binderbauer’s break with the mainstream fusion community.
The ultimate goal of fusion projects like ITER and the Joint European Torus in Oxfordshire, England, currently the largest operational fusion plant, is to capture the tremendous energy liberated by the merger of deuterium and tritium and put it to practical use. The plants would convert the raw fusion energy into heat, then use the heat to boil water, spin a turbine, and ultimately to generate electricity that can light your home and charge your phone. The fusion reaction is so hot that no physical object can contain it. Instead, researchers hold the fuel in place using magnetic fields—typically a donut-shaped field configuration called a tokamak. Magnets alone account for much of the tremendous bulk of a fusion plant like ITER.
But the real action in a deuterium-tritium plant, as is true for all fusion energy, takes place at the atomic scale. The nucleus of regular hydrogen is just a single proton. Deuterium is a proton bound to a neutron, the electrically neutral cousin of a proton. (Adding a neutron to an element makes it more massive but does not alter its chemical identity.) Tritium is a proton bound to two neutrons. When deuterium fuses with tritium, the end product is helium—two protons bound to two neutrons—plus a spare neutron, which goes flying off at high speed. Deuterium and tritium nuclei both carry an electric charge, which means they can be held in place with magnetic fields. Neutrons offer no such means of control. They are like cats: They do what they want.
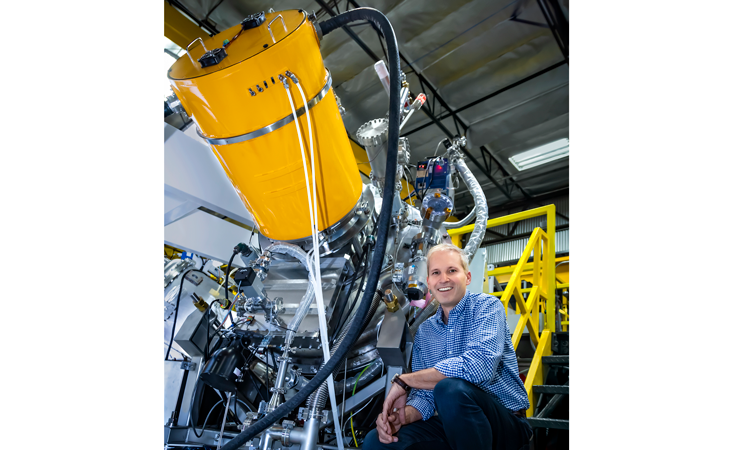
Neutrons carry up to 80 percent of the energy in deuterium-tritium plants, so the plants will be designed to capture the neutrons and absorb their energy in a surrounding solid or liquid blanket. Some neutrons can escape, though, siphoning away energy. Those strays can embed themselves into surrounding parts of the reactor, turning materials brittle and radioactive.
Deuterium-tritium fusion proponents see this “neutron flux” as an eminently solvable technical issue, one they’ve been aware of for decades. “We don’t have the right materials right now. They’ll need to be tested and validated, and it’s a long path, but we’re working on them,” says Tony Donné, program manager for EUROfusion, a research consortium that supports ITER. Those optimized materials should allow ITER to run for 15 years before it will require neutron-related maintenance, he expects. Bob Mumgaard, CEO of Commomwealth Fusion Systems, regards neutron flux as part of a fusion power plant’s wear-and-tear—a routine aspect of its servicing cycle, happening perhaps once or twice a year. “We can simplify the internal components, develop maintenance scenarios,” he says. “We have such a scheme substantially in place.”
The firestorm that ensued was overwhelming and scary.
Rostoker regarded neutron flux as a potential showstopper. The amount of radioactivity created inside a deuterium-tritium plant would be less than what is produced by a nuclear plant, Binderbauer notes, but it could still be enough to present thorny economic, political, and regulatory issues. In an email, Mumgaard points out, “The level of radioactivity generated by neutrons is low, comparable with a medical test facility. The half-life of materials activated by neutrons is thousands of times lower than radioactive waste from a fission nuclear reactor.”
Binderbauer has another worry about deuterium-tritium fusion. Tritium is radioactive, he notes, with a half-life of just 12.3 years, meaning it needs to be handled and monitored with the same kind of caution as any other radioactive material. It is also chemically identical to the light hydrogen found in ordinary water. “If it gets in the groundwater and you drink a glass of water with tritium in it, you’ve got a 12-year time bomb ticking away inside you,” Binderbauer says. (A fact sheet from EUROfusion notes that “tritium poses a serious threat to human beings if it replaces hydrogen in our biological systems.”) Nuclear weapons facilities have developed detailed procedures for handling tritium, which is encouraging or unsettling, depending on your perspective.
“I hope this doesn’t come across too negative,” Binderbauer adds unconvincingly. Rejecting the mainstream approach to fusion was integral to his formative work with Rostoker and all that has followed from it.
Step one in Rostoker’s and Binderbauer’s quest was coming up with a fusion reaction that could replace deuterium-tritium. In 1994, after weighing the options and consulting with their colleague Hendrik Monkhorst at the University of Florida, the two identified a far more plausible option: a fusion reaction between a proton (a hydrogen nucleus) and the element boron. The end product of their union is three helium nuclei, with just a trace of nuclear byproducts. Proton-boron fusion eliminates more than 99 percent of the neutrons produced by deuterium and tritium (although that still leaves enough neutron flux to require careful shielding). As for the fuels themselves, they are about as harmless as you could hope for. Boron is mined as the common mineral borax, and is widely used in building materials, glass, and detergents. Hydrogen is the predominant element in every glass of water.
“I wouldn’t say we fell in love, exactly, but we got convinced we could really make it work,” Binderbauer says. “Now you’ve got almost no neutrons, and you’ve got a plentiful terrestrial fuel. There’s no radioactive input or output in the primary reaction. The helium that comes out is completely innocuous. It was a beautiful thing.”
The less beautiful thing was trying to figure out how to make proton-boron fusion actually happen. Igniting proton-boron fusion requires temperatures around 3 billion degrees Celsius, roughly 30 times hotter than deuterium-tritium fusion. Nobody had yet figured out how to get an energy gain using the easier reaction. Moving on to an even harder problem would be madness, Binderbauer understood, unless he could find a new, more effective technological approach. “We couldn’t look within the box of already available concepts because they all were handicapped,” he says. The tokamak is the best-studied approach to making fusion happen, but it is insufficient for the extreme demands of proton-boron fusion.
To meet those demands, Binderbauer and Rostoker developed a radically different design for a fusion reactor, one resembling a particle-physics experiment much more than a tokamak. Instead of a magnetic donut, they would use a tube. Instead of trying to hold onto the fuel from the outside, they would spin it so that it would create its own magnetic field from the inside—a “field-reversed configuration” in the lingo of the business. And to get the process started, they would crash together beams of protons and boron nuclei, much as physicists do at huge particle-accelerator facilities like the Large Hadron Collider.
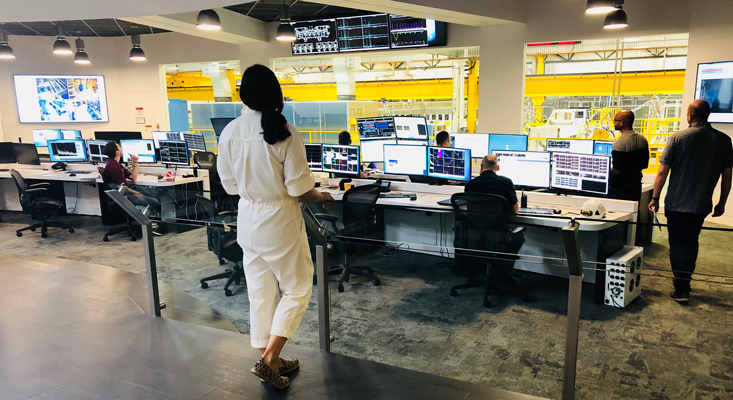
The obvious next step was to start building devices that could put these ideas to the test. “We had exhausted what we could do on the cheap. We wanted to instigate getting federal support,” Binderbauer says. So in 1997, he, Rostoker, and Monkhorst wrote a paper outlining their findings and managed to get it accepted by Science.
He wanted to cause a stir, and succeeded a bit too well. Science was inundated with critical letters. “We were naïve about thinking that people would say, ‘Well here are the shortcomings of the tokamak, here’s a smart alternative, here a machine that may be able to do a non-neutron reaction’,” Binderbauer recalls, shaking his head at his younger self. “We didn’t anticipate the firestorm that ensued. It was overwhelming and scary.”
Judging from the letters, some of the critics either didn’t understand the paper or didn’t want to believe its conclusions. Much of the fury, Binderbauer now realizes, came from colleagues who felt like their careers were on the line, and not without justification. The Science paper came out just as the U.S. Department of Energy was cutting back on fusion research. The last thing people in the field wanted to see was some upstart vying for some of that scarce funding and implying that the standard approaches to fusion might be a dead end.
Binderbauer had already walked away from all of the physics and engineering work supporting deuterium-tritium fusion. Now he realized he would have to walk away from the government spending that had supported fusion research since the 1950s. “This is where we sobered up,” he says. “I told Norman, ‘Look, we’re not going to get federal dollars. Why don’t we try private?’”
Up to that point, there had been only one notable private investment in fusion energy. In the late 1970s, Penthouse magazine founder Bob Guccione sank $17 million into a project to build a miniature fusion machine that snarky scientists nicknamed the Porno-mak. “So we’re not the only crazy ones to try this,” Rostoker responded dryly.
With little to lose, he and Binderbauer took the plunge and incorporated—first as Colliding Beam Fusion Reactor, Inc., later as Tri-Alpha Energy, and finally as TAE. Their early efforts attracted an unusual alliance of supporters, including Nobel laureate Glenn Seaborg and actor Harry Hamlin, best known as the “sexiest man alive” actor from L.A. Law. After a proposed $15 million investment from Texaco fell through at the 11th hour, they managed to scrounge up only about $1 million, far too little to launch a fusion research lab. They drastically scaled back their ambitions and set out to build a small, proof-of-concept experiment called “the sewer pipe.” It was built from, yes, a sewer pipe, wrapped with magnets and electronics designed to create the hoped-for field-reversed configuration.
Ratty as it looks, the sewer pipe experiment produced exactly the kind of magnetic field it was supposed to, the first tangible evidence that Binderbauer was not nuts. It is still on display at TAE. If the company succeeds, it may be remembered as the icon of proton-boron fusion.
Not many others in the fusion community see that as a smart wager.
Once TAE could demonstrate that they had a viable concept and a team that knew how to execute it, more investors began to sign on—among them the late Paul Allen, co-founder of Microsoft. That funding allowed the company to create a proper testbed for a field-reversed fusion reactor, called C-2. From there, C-2 expanded into a bigger machine called C-2U, which in turn evolved into TAE’s current monster of an experiment, C-2W.
After C-2W was completed in 2017, Binderbauer renamed it “Norman” in honor of Rostoker, who had died on Christmas Day, 2014. Norman recently completed its primary research goals, including achieving temperatures above 30 million degrees Celsius and keeping the plasma stable for 30 milliseconds—hot enough and long enough to validate that everything is working as expected. Most important, Norman validates the theory that a field-reversed reactor can be scaled up, reaching conditions beyond what is possible in a tokamak. At ultra-high temperatures, tokamaks can become leaky and difficult to maintain; a field-reversed configuration should become more stable. TAE is betting that the theory will hold true all the way to the 3 billion degrees needed for proton-boron fusion.
Not many others in the fusion community see that as a smart wager. Donné of EUROfusion, who has spent a career working with tokamaks, admires TAE’s grand ambitions but is wary of the company’s reliance on as-yet unproven physics and engineering. “You need to get orders of magnitude higher in temperature. If you go with that direction in fusion, it will add a half a century to the timescale,” he predicts.
For 20 years now, the TAE team has repeatedly braced for failure but then managed to barrel through another seemingly impassible physics barrier, says Artem Smirnov, TAE’s chief technology officer—the dour yang to Binderbauer’s cheery yin. Few people thought the original sewer-pipe experiment would work. Few people thought that a field-reversed reactor like Norman could achieve stable, 30-million-degree heat. “But with every step we take, there’s new uncertainty,” Smirnov admits. “What if the scaling law fails somewhere along the way? We can’t be sure until we get there.”
The next step in that journey is the $200 million Copernicus reactor, intended to reach temperatures around 100 million degrees. TAE is currently real-estate shopping in Orange County, with the goal of breaking ground this year and beginning test runs in 2023. Copernicus should be powerful enough to ignite deuterium-tritium fusion, but that is not its job; its real purpose is to scout out those unknown regions of high-temperature physics. If TAE’s take on the field-reversed reactor design keeps delivering as expected, then Binderbauer will go all-in on his biggest bet of all: a “two-ish-billion-dollar” proton-boron fusion reactor called Da Vinci. At last, it should hit the magic 3 billion degrees, keep a proton-boron fuel stable, and produce a healthy output of fusion energy.
Da Vinci is intended to mark TAE’s transition from fusion prototypes to commercial fusion energy. It will not be powering anyone’s home, but it will be designed with electricity generation and mass production in mind. From then on, TAE will be relying heavily on companies like General Electric that have deep experience in designing and constructing power plants. “More important than the money is the industrial-scale support,” Binderbauer says. “You’re not building just one machine. You’re thinking about all the next machines, and they should be built by the industrial sector, not by TAE.”
Binderbauer’s roadmap to his kids’ energy future looks like this: The first commercial-design fusion reactor begins operation in the early 2030s. Over the next 10 to 15 years, fusion captures a few percent of the global electricity market. Companies start up production lines to crank out modular fusion reactors. Governments might provide incentives for carbon-free energy, whether in the form of taxes or subsidies, speeding the growth of the new industry. Wind and solar energy continue to expand, but fusion expands, too, balancing them out. “In the second half of the century, would fusion capture a large fraction of the market, maybe the dominant fraction? I would not be surprised,” Binderbauer says.
That would be a striking validation of Rostoker’s original vision, even if TAE does not end up being the company to bring practical fusion energy to market. Rostoker’s determination to pursue an unconventional scientific approach, followed by his decision with Binderbauer to rely on private funding, have already had a tremendous ripple effect. There are now more than a dozen startups pursuing fusion plans. “Sometimes in life, curveballs come your way and you just need to have enough intuitive sense to grasp them,” Binderbauer says. “Working with Norman was the best decision I ever made.”
He still takes inspiration, too, from one of his last interactions with Rostoker. In the fall of 2014, Binderbauer delivered a fusion-energy presentation to a packed auditorium at a meeting of the American Physical Society—a sweet vindication after his pariah status in the late 1990s—and wanted to share the good news with his mentor. By this time, Rostoker was in the advanced stages of dementia and was unable to process the story or to recognize his friend. Even so, he could access enough memory to point to a framed photo on the wall, showing the TAE staff gathered around the C-2 reactor. Binderbauer recalls the moment vividly. “‘You know what this is?’ he asked me. And I said, ‘No, tell me Norman, what is it?’ He said, ‘This is TAE, that’s the company I started and that one of my best students runs. We’re going to go the distance. We’re going to make economically successful fusion. And it’s going to change the world’.”
Binderbauer looks at me with an opaque mix of overlapping emotions. “It’s one of the saddest stories, but also a really beautiful story. It’s the triumph of an idea.”
Corey S. Powell enjoys exploring the outer possibilities of physics and astronomy. He writes the Out There blog and co-hosts the Science Rules podcast. @coreyspowell
Lead image: Courtesy of TAE Technologies