What is orbital rotation? The basic picture is clear enough: One body is at rest, while the other follows some circular or elliptical path around it. The trouble is just to figure out which body is which. If you’re standing on the surface of the earth, it appears that the sun slowly orbits around you once per year. (Of course, it also looks as if the entire sky rotates around you once per day, because the earth rotates on its axis. But we are thinking about the motion of the sun relative to the rest of the sky, which happens on a yearly cycle.) But on the surface of the sun, it would presumably also look as if the earth orbits around you once per year. Figuring out which of these reflected the true motions of the solar system, as opposed to merely apparent motions, was once a matter of heated controversy.
Seventeenth-century physicist Galileo Galilei, for instance, was convicted of heresy for claiming that the earth orbits around a stationary sun. He spent the end of his life under house arrest. Even so, he had it better than Giordano Bruno, who in 1600 was burned alive for his heretical views, which included the belief that the earth moved.
We all know how this story ends. The key was provided by Isaac Newton, whose late 17th-century masterpiece, Mathematical Principles of Natural Philosophy, was written to settle, once and for all, the true motions in the solar system. To do this, Newton produced a novel theory of space, time, and motion, along with his famous theory of universal gravitation, which explained how the sun, planets, and other bodies in the night sky influenced one another’s motion. His theory showed that neither the sun nor the earth was at rest: Both orbited around the center of mass of the solar system. But since the sun is so massive, the center of mass of the solar system is very close to the center of the sun, and so, for all practical purposes, Galileo and Bruno got things right.
There is nothing in general relativity that quite answers to the description “orbital rotation.”
At least, they got things right insofar as Newton’s theory was correct. Today, physicists think that Newtonian gravitation is merely a useful approximation to general relativity, a different theory of space, time, and gravitation that Einstein developed about a century ago. It turns out that orbital rotation in general relativity is much more subtle than Newton, or anyone else before the 20th century, imagined. In fact, David Malament, a philosopher of physics at the University of California, Irvine, has shown that there is no notion of rotation in general relativity that lives up to the basic picture sketched above. (Full disclosure: Malament is my colleague at UC Irvine, and before that, he was my Ph.D. supervisor.)
Suppose you wanted to test whether a body is rotating something. For Newton, there were some simple experiments you might do, any of which would suffice. For simplicity, think about a circular ring, rotating (or not) around an imaginary axis passing through the center of the ring, perpendicular to its plane (basically, a bicycle wheel rotating around the axle, though without any connecting spokes). Because it’s a ring, you don’t need to worry about different speeds at different parts of the object, like with a sphere. (It’s almost always simpler to think about lower-dimensional cases.) Yet it will turn out that even in this simple case, there are basic ambiguities in general relativity concerning whether the ring is rotating or not.
Here is one experiment to test if the ring is rotating. Suppose you are located on the axis at the center of the ring. Take a telescope, point it at the ring, and check if the ring appears to move. Of course, for this to work, you need to make sure that you and your telescope are not rotating about the same axis, since in that case the ring might appear to be rotating even if it isn’t. You can confirm this with a simple test, originally proposed by Newton: Take a bucket of water—if the surface of the water is perfectly flat, then the bucket is not rotating, since the water would rise near the edges of the bucket and depress in the middle. If your telescope is not rotating relative to the bucket (say, because you have glued the telescope to the top of it), then you can conclude that the ring really is rotating if it still appears to move.
You might also try to test for orbital rotation by doing experiments on the ring itself. One way to do this would be to set up a series of mirrors around the ring so that you could shine a laser and have the mirrors bounce the laser around it. Suppose you were to do this twice, shining the laser in the two opposite directions around the ring, and then measure the round-trip time in each direction. If the ring is rotating, then light going around in one direction should have to travel less distance than light traveling in the opposite direction, because the ring would have moved in the same direction as one, but not the other, beam of light. Devices that measure rotation in this way are known as Sagnac interferometers. They are highly sensitive and are in widespread use in navigation.
Yet a third way to measure orbital rotation would be to use a gyroscope, which is a wheel mounted on a base in such a way that the wheel is free to spin about an axis that itself can move around relative to the base. A gyroscope measures changes in orientation, because once a wheel starts spinning around some axis, it tends to continue spinning around the same axis, even if you move the base of the gyroscope around. They are used in aircraft, for instance, to measure changes in a plane’s attitude, and in smartphones to detect motion of the phone. A gyroscope can similarly be used to determine if our ring is rotating, as follows. Attach the base of the gyroscope to the ring, and set the wheel rotating about an axis tangent to the ring. If the ring is rotating, then the axis of the gyroscope wheel will move relative to the base, since the base will rotate along with the ring. If the ring is not rotating, then the axis of the gyroscope would remain stationary relative to the base (and the ring).
In Newtonian physics, all three of these tests would always agree. After all, they all measure the same thing: orbital rotation. But in general relativity, no two of these tests agree in all cases. This is a strong hint that that there is nothing in general relativity that quite answers to the description “orbital rotation,” at least as we are used to thinking about it.
The key to understanding this lies in how Einstein reimagined inertia. To Newton, inertia was a body’s tendency to move in a straight line at constant velocity unless acted on by some outside force, such as gravity. Orbital rotation is necessarily a non-inertial form of motion, because orbits are not straight lines. The situation in general relativity is quite different. There, instead of deflecting bodies from their straight-line, inertial trajectories, gravity acts by distorting the straight lines, in a way that depends on the distribution of mass and energy in the universe. This distortion is known as space-time curvature. Rather than follow straight lines by default in general relativity, bodies travel on curved lines or, put another way, on straightest lines in curved space and time.
These changes in how inertia works can have strange consequences. For instance, if a massive body, such as the sun or a black hole, is spinning, it twists space and time around with it, breaking the Newtonian link between inertial motion and straight-line, non-rotational motion. This phenomenon is known as frame-dragging, and it has been tested experimentally using satellites orbiting earth.
Does this mean that the earth does not orbit around the sun? Not so fast. As it happens, Newton’s theory provides an excellent approximation to general relativity in our solar system, where the effects of frame dragging are very small. So for all practical purposes, it is still appropriate to say that the earth orbits the sun. But what Malament’s results do show is that rotation is a fragile concept. There are more extreme cases, such as a spaceship moving near a spinning black hole, where it just doesn’t make sense to ask whether the ship is orbiting the black hole. In such cases, the black hole can distort space and time so severely that the very concept of rotation breaks down.
James Owen Weatherall is Professor of Logic and Philosophy of Science at the University of California, Irvine. His most recent book is Void: The Strange Physics of Nothing (Yale University Press, 2016), which explores the structure of empty space in physics, from the 17th century to today. His website can be found at jamesowenweatherall.com.
Watch: The Princeton physicist Paul J. Steinhardt discusses whether we can know the universe’s past.
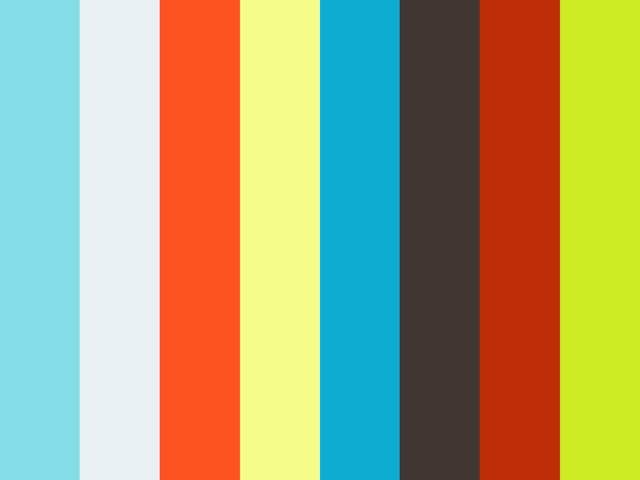
The lead image is courtesy of NASA’s Marshall Space Flight Center via Flickr.