This classic Facts So Romantic post was originally published in June, 2013.
Take a look at a butterfly’s wing, and you can learn a lesson about life. Not that it’s beautiful, or fragile, or too easily appreciated only when it’s fading—though all that is true, and evident in a wing.
Look very close, at the edge of a pattern, where one color turns to another. The demarcation isn’t so abrupt as it seems at arm’s length. It’s not a line, but rather a gradient.
This is a lesson about uncertainty.
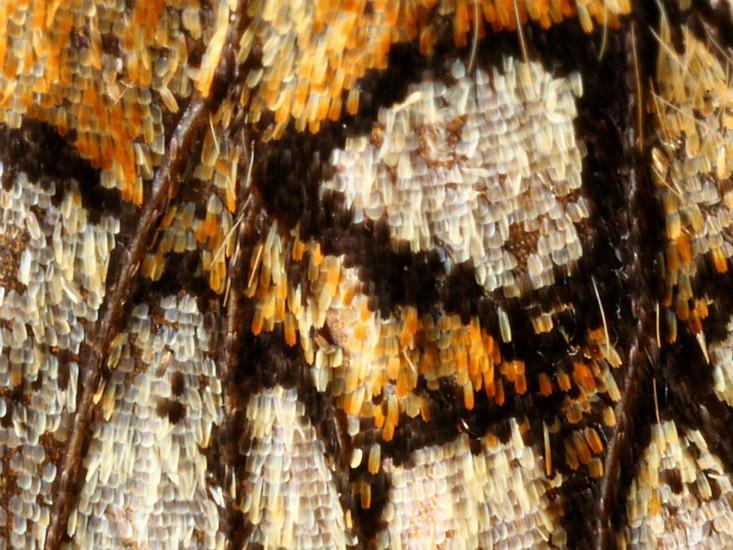
A butterfly’s colors come from its scales, each a single cell, pigmented a single hue. At pattern boundaries, scales of different colors intermingle. Transitions and shading are achieved by varying the proportions of the mix. It’s beautiful. It is also, in the language of molecular biology, a model for a stochastic mechanism of gene expression.
Each scale’s fate is not preordained. Cells on the surface of a swallowtail’s wings, for example, were not originally specialized to be yellow or blue or black. Instead they contain genes potentially capable of producing each of those pigments.
What determines the color of each butterfly scale is, in a word, chance. A molecule hits a piece of cellular machinery at just the right moment, in just the right place, and a gene produces a certain pigment. There’s no guarantee it will happen. It’s a matter of probability and moment-to-moment randomness. (The same probabilistic mechanism underlies the portions of the wings with solid colors, too. In those parts, molecules that trigger genes for one color are present at such high concentrations that the final color outcome is assured.)
What determines the color of each butterfly scale is, in a word, chance.
In biology, there’s a tendency to conceive of randomness as noise, an accidental factor, a product of error—random genetic mutations, for example, are mistakes in a chromosome-duplicating system that’s supposed to make perfect copies. Random mutations might be harmful, or insignificant, or beneficial, but they’re fundamentally mistakes, a disorderly deviation from an orderly system.
What makes a butterfly’s wings so remarkable isn’t just that unpredictabilities underlie their colors, but that they’ve harnessed the probabilities.1 Randomness and uncertainty are translated into the ordered, functional patterns of a monarch or checkerspot. And in this, the butterfly’s wing is not unique, but a manifestation of principles ubiquitous in biology.
Let’s put on our Powers of Ten goggles and increase our magnification to where cell activity occurs, the level of so-called cellular machinery. We’ll have to abandon that metaphor, though: Cells indeed contain complicated, task-performing structures, but the word “machine” is a product of the macroscopic world. We think of machines as rigidly assembled, with predefined purposes. At the cellular level, the analogy falls apart.
Out at the leading edges of theoretical and computational and experimental biology, where known and unknown meet, cellular machines have been redefined.2 The proteins of which they’re made don’t fold and unfold and operate according to some stepwise blueprints. Shape and function are exquisitely sensitive to infinitesimal energetic shifts, to the motion of atoms and the forces they exert.
Rather than a cellular factory, then, imagine a restaurant with a kitchen where blenders turn into convection ovens and whisks into knives when someone walks by, raising ambient temperatures by a fractional degree. Imagine that the whole kitchen is like this, that cooks and prep staff, though they move with intent, can’t help but wander around—and still the seven-course meals come rolling through the doors.
Undoubtedly this metaphor has its own problems, but it gets the point across: The cellular world is an ever-fluctuating place. It’s full of randomness and, when things aren’t narrowly random, with uncertainty. Atoms and molecules and gradients change from moment to moment, and proteins with them.
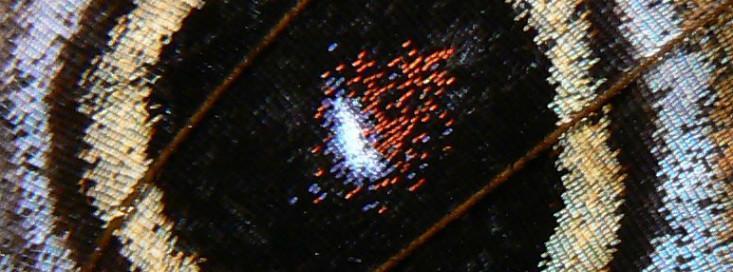
Here one might ask where uncertainty comes from: Is it truly uncertain? Might every molecular fate be predicted, if only we knew the motions and properties of every particle in a cell? Or does quantum physics enter the equation at some level, with all its spooky uncertainties and strange probabilities shaping biology in some fundamental way?
That we don’t know, and might never. It’s a question too hard to study. Whatever the case, certain molecular activities are, best as we can describe them, random or probabilistic. And we know, up at the cellular level, that a cell’s fate—whether an embryonic stem cell becomes specialized for service in kidney or liver, whether a blood stem cell grows up to carry oxygen or identify pathogens—is to some extent stochastic, determined by a signal that may or may not appear.
What’s extraordinary, again, is that from all this uncertainty, form arises. Two identical twins, after trillions upon trillions of cell divisions, actually look the same to our eyes. Out of disorder, order—though of course having identical genomes is no guarantee of identical outcomes. Indeed, stochasticity’s role in cells became evident when genetically matched yeast colonies, raised in the exact same environments, developed in very different ways.
What seems to explain that, it turns out, is variation in so-called epigenetic responses—processes that alter gene activity according to environment and circumstance, allowing organisms to change their biology in response to life’s unpredictable demands. The different yeast colonies had different epigenetics; they responded to uncertainty differently. That might itself have been the product of chance, some “inherited stochastic variation,” though the benefits are obvious. It’s evolutionary bet-hedging, a way of increasing the adaptive possibilities for one’s descendants, despite their genetic similarities.
Again we see biology using uncertainty, building on it, making it integral to life. And it’s evident not only in epigenomes, but in genomes: When we look at our own, which for each of us contain some number of apparently random errors produced by copy-making glitches, we find that the errors are not randomly distributed. Mutations occur at different rates in different parts of the genome.
This isn’t the same thing as saying that certain sequences tend to stick around over evolutionary time, because errors there are more likely to cause problems. Instead, the potential for a random error to occur in the first place fluctuates across the genome. At every cellular level, randomness is harnessed.
That we don’t know, and might never. It’s a question too hard to study.
“Life is a study in contrasts between randomness and determinism,” wrote biochemists Arjun Raj and Alexander van Oudenaarden in an article entitled, “Nature, Nurture or Chance,” in the journal Cell. “From the chaos of biomolecular interactions to the precise coordination of development, living organisms are able to resolve these two seemingly contradictory aspects of their internal workings.”
Do these resolutions occur at even higher levels? Pattern from chance in populations, species, communities, ecologies? In our own lives? We can’t look at societies or lives the way we do cells, but certainly we feel it, at some intuitive level. “I think back on the trajectory of my life, and I think: I happened to bump into this person on the train, and it led to this or that,” Raj told me. “So many things are unpredictable on a long time scale, though it feels like they are predictable in the moment.”
I think on my own life: My parents met on a train. My closest friends came from chance encounters in a subway station, a class, a hockey team, a writing assignment. I can’t imagine my life without them, yet each of those meetings was profoundly, unsettlingly improbable. And why stop with friendships? Why not scale up to the level of the universe itself, where order and disorder interpolate in random patterns?
There, perhaps, from the perspective of God or some alien cosmologist or deep time or whatever you use to imagine inconceivable vastness, we might find order yet again. Who knows for certain; we likely never will. But we can look at a butterfly’s wing and wonder.
Footnotes
1. The pigment-synthesizing genes that guide coloration in a butterfly’s wing are an ideal model system because they’re relatively simple and straightforward. That’s the exception, not the rule. Most traits involve multilayered cellular and genetic relationships: networks of interactions nestled inside networks of interactions, often behaving in nonlinear ways, a whole biological nesting doll of complication. Which makes their harnessing of randomness and uncertainty all the more extraordinary.
2. There’s a certain amount of uncertainty to these descriptions. It’s fairer to say, some scientists who study these matters think this is happening, and the fragments of biochemical data we’re able to retrieve at molecular and atomic scales inside cells match our computational models, but it’s slow going.
Take, for example, the modeling of protein folding and unfolding described in this PNAS article, produced by a custom-designed, massively parallel piece of dedicated hardware that’s roughly 100 times more powerful than any other machine used for this purpose. Running at full power over the course of a day, it can model a scant ten microseconds of molecular cell dynamics. Run it for 2,737 years, and you’d describe one second.
Brandon Keim is a freelance journalist who writes about science, technology, and nature. His work has appeared in Wired, Aeon, Scientific American Mind, and other publications.