Some dark, clear nights, when the blazing stars cast shadows down on Mauna Kea, Hawaii, the astronomer Olivier Guyon steps away from his workbench and computer screens and walks outside the giant 8-meter Subaru Telescope to savor the heavens. Guyon began his habit of stargazing about the same time he first decided to become an astronomer, as a young boy in the countryside of northeastern France. At 17, he built his first telescope, a half-meter Dobsonian he still occasionally uses today. Guyon kept stargazing through college, then graduate studies at the University of Paris and doctoral work at the University of Hawaii, but today, in his late 30s, with waves of silver lapping at the edges of his dark hair, he can rarely spare the time. He steals a glance skyward, eyes large, and for a moment seems to be a boy again, starstruck for the very first time, before lowering his gaze and walking back inside to become a full-grown astronomer again, too consumed by his work for casual stargazing.
As an astronomer, Guyon’s tether to Earth has always been tenuous, but it has grown more threadbare ever since he began splitting his time between the Subaru telescope in Hawaii and the University of Arizona in Tucson, where he is a professor. Some weeks he spends more time in the air than on the ground, flying back and forth across the Pacific. If he ever gets jet-lagged, he hides it well enough, and his employers consider his work so valuable they don’t complain about the cost and trouble of his transits. Colleagues on both sides of the ocean know him for wearing Hawaiian shirts and an easy smile, as well as for his curious tendency to be both taciturn and talkative. Strike up a conversation about the weather and Guyon can tend toward the monosyllabic, but delve into his work, and his French-accented words begin to stream forth in languid succession like water-polished pebbles tumbling along a riverbed.
In a 2012 interview with the MacArthur Foundation, after it awarded him one of its $500,000 “genius” fellowships, Guyon characteristically gushed that “when you look at the sky at night, every star you see is another Sun.” His capitalization is implicit, an honorific to communicate the profound reality that each scintillating stellar point overhead is, just like our own sun, a locus of light and heat for swarms of planets, orbiting as yet unglimpsed. To date, astronomers have only directly imaged about a dozen planets beyond our solar system, each far too large and intemperate to closely resemble Earth or support life as we know it. Yet of all the planets in their billions still unseen, some could perhaps be very much like the Earth, orbiting in their stars’ “habitable zones,” circumstellar regions neither too hot nor too cold for life as we know it.
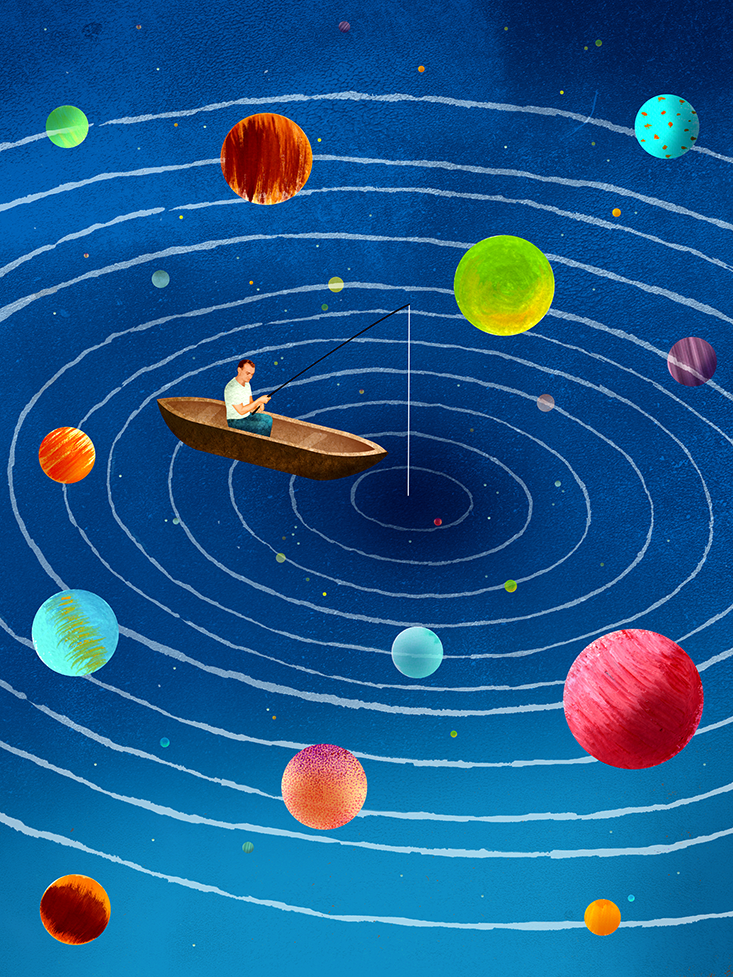
That we don’t yet see these worlds is because planets such as ours are so very faint and so very close to their much brighter and bigger parent stars. Imaging and studying them around a representative sample of nearby stars, it has been thought, would likely require a very large space telescope, one with a light-collecting aperture perhaps 8 meters in diameter, high above our planet’s distorting atmosphere. No one can say for certain how much such a telescope would cost, but common estimates of $5 or even $10 billion have placed such projects on space-agency backburners until, optimistically, the 2030s.
Like Subaru and the University of Arizona before them, the MacArthurites came calling because Guyon is at the forefront of a slow-simmering revolution in optical science that could reveal some of these hidden worlds using space telescopes roughly half the size—and thus, it is hoped, half the cost. Indeed, if the majority of nearby stars harbor rocky planets in their habitable zones, Guyon’s work could provide a pathway to even lower costs, allowing a coming generation of extremely large ground-based telescopes to image some of the closest candidates and study them for signs of habitability and life.
“My goal is to show everyone that it doesn’t have to take decades to do this,” Guyon says. “We can actually image and study some potentially habitable planets fairly soon. We should be working on the technology and planning to make this possibility a reality.”
Unlike other astronomers who subsist on starlight like manna from heaven, Guyon seeks to ruthlessly suppress it, to annihilate a star’s light with exquisite optical violence.
If you can picture yourself perched on a rooftop in New York City, peering through a telescope aimed at a gnat fluttering next to a streetlamp in Los Angeles, you might grasp the difficulty of glimpsing a mirror Earth against the 10-billion-times brighter glare of the million-kilometer-wide fireball that is an alien sun. That 10-billion-to-1 contrast ratio means capturing just one photon of planetary light is rather like sifting through the entire human population of Earth in search of a single person who can tell you some secret of the world. Because a mirror Earth is so very faint, even if you did somehow manage to capture each and every photon reflected off its distant lands, seas, and skies, they would arrive so intermittently at your telescope that you could practically tally them off by counting with your fingers. The resulting image would be unimpressive—an unresolved dot of planetary light—but it could still be used to map hemisphere-scale surface features and to study a world’s atmospheric composition.
And so, unlike other astronomers who subsist on starlight like manna from heaven, Guyon seeks to ruthlessly suppress it, to annihilate a star’s light with exquisite optical violence so that any accompanying planets may be seen. His weapon of choice is something called a coronagraph, a device built into a telescope that occludes a target star’s light in much the same way you might blot out the sun in the sky with your thumb—covering it in the sky and casting a shadow toward your eyes. Relatively simple coronagraphs capable of millions-to-one contrast ratios have been available since the 1930s, but when the hunt for mirror Earths began in earnest last decade, instrumentalists began devising a host of more ambitious and demanding designs.
Crafting a coronagraph to achieve a 10-billion-to-1 contrast ratio is a rather impractical pursuit. Other than taking snapshots of small planets orbiting other stars, no other application has yet been conceived that requires reaching such extreme contrasts—developing the technological capability to do so is thus purely a matter of satisfying curiosity. Freed from any commercial considerations, the frontier of coronagraphy can be seen as a bit metaphysical, an almost-gnostic quest for reconciliation between light’s timeless purity and the ephemeral corruptions of matter.
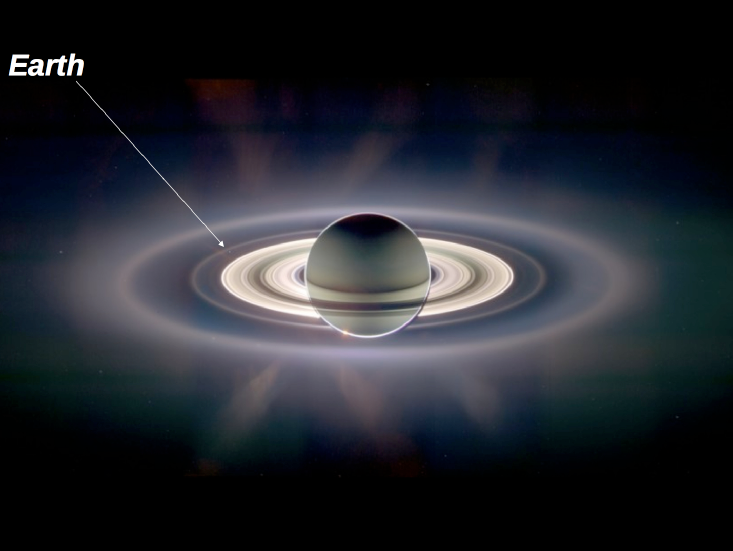
Inside a coronagraph, light doesn’t really behave as the particulate shower of photons you might expect. Instead, it acts more like a fluid, diffracting and flowing in wavelets across and around mirrors and other optical components. When the perfection of a beam meets even atom-scale flaws in the shapes and positions of those components, the light scatters and shifts its trajectory, leaking elsewhere in the telescope. All too often the stray photons splash upon world-seeking sensors, contaminating any resulting image with glinting speckles that can obscure or masquerade as legitimate planetary light. Even a perfect flat mirror cannot be immune: Starlight smoothly propagating across a flawless surface still diffracts when it reaches the mirror’s edges, piling up in patterns of shimmering rings that are at least as bright as dim planets.
Over time, astronomers have learned to fight these diffractive rings by preventing a beam of starlight from encountering edges at all. One of the simplest and most common ways to suppress the diffractive rings is a process called “apodization,” which involves somehow making a beam’s perimeter darker than its center, forming an insulating barrier of darkness against diffraction. Early apodized planet-finding coronagraphs called for simply spray-painting a darkening gradient out to opacity from the center of a mirror, or masking a mirror’s edges with opaque overlays custom-cut in computer-generated patterns. Some of these designs worked well enough on paper and in basic laboratory tests to image a mirror Earth, but they were all relatively inefficient, reducing a final image’s resolution and discarding a great deal of light from stars and any accompanying planets alike. Worse, to sufficiently suppress starlight close to sun-like stars to see what sits in their habitable zones, any space telescope using the coronagraphs would require a very large and very expensive mirror, something on the order of several meters in diameter.
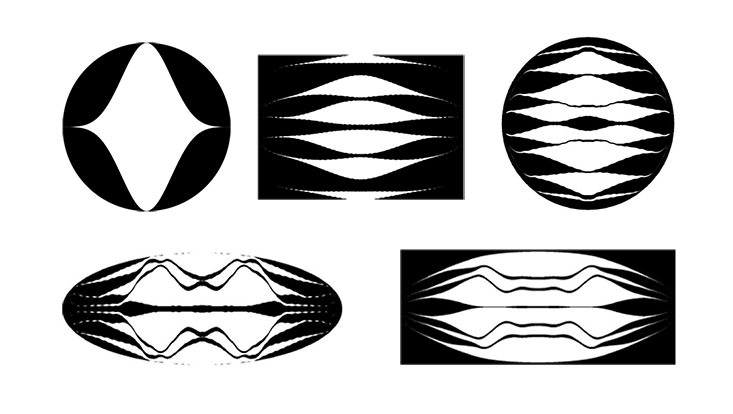
Kasdin, N.J., Vanderbei, R.J., Spergel, D.N., & Littman, M.G. Extrasolar Planet Finding via Optimal Apodized-Pupil and Shaped-Pupil Coronoraphs. Astrophys. J. 582 1147-1161 (2003).
© AAS. Reproduced with permission.
All this was on Guyon’s mind in the late summer of 2002 just after obtaining his Ph.D., in which he had studied multiple methods to directly image planets. He was visiting an observatory in Victoria, British Columbia, doodling with pencil and paper on a quiet misty morning while many of his colleagues were still sleeping. “I remember thinking about how inefficient [those designs] were, and wondering if I could achieve the same apodization effect by somehow moving the light around, by concentrating it into the center of a beam instead of just throwing it away at the edges,” he recalls. Guyon began drawing sets of concentric rings that could be used to mask a mirror’s edge and shape a beam. The breakthrough came when he began connecting the rings together, sketching out continuous surfaces: Instead of masking a flat mirror’s edges to minimize diffraction patterns, he realized he could achieve the same thing without masks at all, by geometrically sculpting the mirror itself. Astronomers had already used the concept in radio telescopes, and, unbeknownst to Guyon, optical scientists had recently devised similarly aspheric mirrors to alter the shapes of laser beams. Guyon’s breakthrough was to propose and develop the technique as an ideal solution for the problem of imaging planets.
The result was a pair of strangely aspheric shapes, each gently curved at its center and flaring into flatness at its edges. Guyon pictured a beam of starlight being stretched like a piece of rubber as it bounced between them, becoming concentrated at its center and diluted at its edges by the interplay of flatness and curvature. The resulting beam, he suspected, would be apodized and free of planet-swamping rings, all while preserving as much planetary light as possible. Focusing the apodized beam with a lens would further sharpen the central starlight into a tiny point that could then be blocked by a simple opaque disk, allowing any faint planetary photons lurking in the beam’s fuzzy edges to stream through unscathed to impact a detector. This combination of high throughput and resolution meant that a planet-finding telescope using Guyon’s coronagraph could potentially be made smaller and cheaper than many other designs while still providing superior performance. Instead of requiring hours or days to produce a useful image of an Earth around a nearby star, Guyon’s method might obtain an equivalent image within minutes.
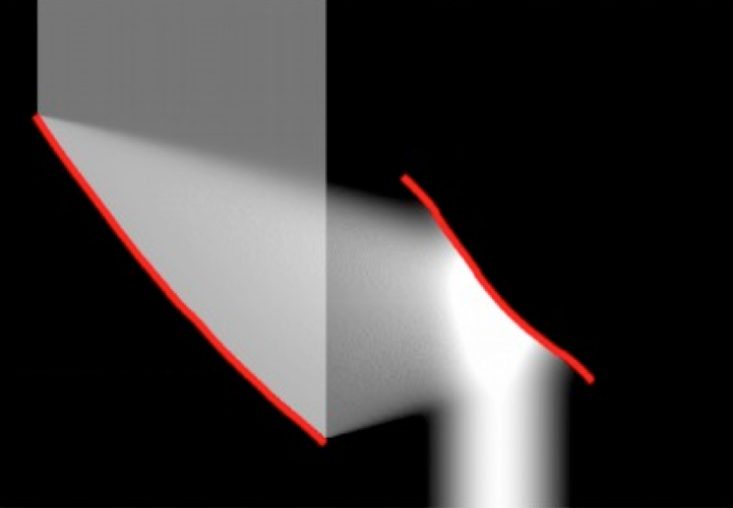
After describing his design in a 2003 paper, in subsequent studies Guyon and several collaborators applied the same technique to lenses as well as mirrors, derived equations describing the required aspheric geometries, and proposed using his coronagraph design within future planet-finding telescopes. Two other researchers, Wesley Traub and Robert Vanderbei, found and corrected potentially fatal flaws in the new apodization approach. Guyon dubbed it Phase-Induced Amplitude Apodization, or PIAA (pronounced PEE-AY) for short—an apt-yet-arcane description of the technique’s key breakthrough. (Besides being a master of optical physics, Guyon also has a bizarre penchant for devising clunky acronyms for his creations.)
The invention of an entirely new variety of coronagraph is a rare event in optical science, and PIAA’s unprecedented manipulations of starlight opened up a whole new realm of experimentation and design. Before long, NASA research centers and other institutions were scrutinizing the laboratory performance of several types of PIAA coronagraphs, testing their potential to suppress starlight on the cheap. “What’s important to remember is that you gain science much more rapidly per dollar spent by optimizing your coronagraph than by increasing your telescope aperture, your mirror size, which is very costly,” Guyon says.
During one of our talks, Guyon shows me a heavily pixelated image of what seemed to be a stone splashing into a pool of water, surrounded by concentric ripples. The stone and the ripples are actually the smothered glare from an artificial light source, suppressed with a PIAA coronagraph by a factor of a few hundred million within a vacuum chamber at NASA’s Jet Propulsion Laboratory. A void looms adjacent to the synthetic star and the innermost ripple, a dark patch of deep, 10-billion-to-1 shadow cleansed of 99.99999999 percent of light, precisely where a real sun-like star might harbor a mirror Earth. “If we were looking at a real star here, and there was a small rocky planet in this region, because of PIAA we would essentially receive all of that planet’s light and see it just as sharply as a telescope would allow,” Guyon says.
Guyon believes a PIAA coronagraph could provide snapshots of rocky planets in the habitable zones of a handful of nearby “red dwarf” stars.
This simplified lab simulation delivers performance similar to what a real space-based instrument would need to image a mirror Earth. Unfortunately, the money to build such an instrument is not easy to come by. The technology development for PIAA had been ramping up in the mid-2000s, just when NASA, the dominant funder of space science, was studying a planet-imaging mission using baseline designs that called for the older coronagraphs operating at low efficiencies and requiring large mirrors. But, also tasked with completing the International Space Station, building a fleet of new rockets to replace aging Space Shuttles, and launching the over-budget and behind-schedule next-generation James Webb Space Telescope, the agency deemed the near-term pursuit of a large planet-imaging observatory unaffordable, and in 2006 put the project on indefinite hold.
Planet-finding coronagraphs received a new lease on life at NASA in 2013, when the agency decided to put one on its WFIRST mission, a much cheaper and smaller 2.4-meter space telescope scheduled to launch around the end of this decade. NASA chose to outfit the mission with the less capable but more mature earlier coronagraph designs, but in a nod to Guyon’s work selected his PIAA concept as a backup. In the meantime, Guyon works to lay the foundation for PIAA in space by integrating it with telescopes on the ground, including a technology demonstrator attached to the 8.2-meter Subaru telescope.
Called SCExAO (SKEX-ay-OH), short for Subaru Coronagraphic Extreme Adaptive Optics, the instrument should soon deliver pictures of Jupiter-like planets around sun-like stars, as well as images of circumstellar debris disks being sculpted by the gravitational pull of Saturn-size worlds. But bolted onto the backside of a 30-meter-diameter light-gathering mirror, a souped-up version of SCExAO could do far more. With such mirrors, slated to debut early next decade, Guyon believes a PIAA coronagraph could provide snapshots of rocky planets in the habitable zones of a handful of nearby “red dwarf” stars—stars that are smaller, cooler, and dimmer than our own sun.
“The closest star to our solar system is Proxima Centauri, and it’s of this type,” Guyon says. “If you put an Earth around Proxima Centauri, the planet-star contrast is an order of magnitude less challenging than that for the Earth around our own sun. The bigger challenge is that to be habitable the planet must be so very close to this small star, and a PIAA coronagraph will help us look close-in where we need to for that. These are the sorts of potentially habitable planets that we will image from the ground in the next decade.”
Beyond that, Guyon’s ultimate motivation is amply clear. “Maybe in the very distant future, we’ll get resolved images of planets that remind us of Earth. Why not? That’s what really excites me about working on this.”
Freelance writer Lee Billings is the author of Five Billion Years of Solitude: The Search for Life Among the Stars.