Most people have never heard of the mouse hepatitis virus (MHV). It’s not the kind of virus that makes the headlines. It has never caused a pandemic. It doesn’t even make people sick. And although MHV can be troublesome for research labs that study rodents, it doesn’t trigger huge economic losses by attacking crops or livestock.
In fact, MHV seemed like such an unpromising subject 40 years ago, when virologist Susan Weiss, PhD, of the University of Pennsylvania decided to start studying it, that her colleagues wondered why she wanted to investigate such an obscure and irrelevant virus.
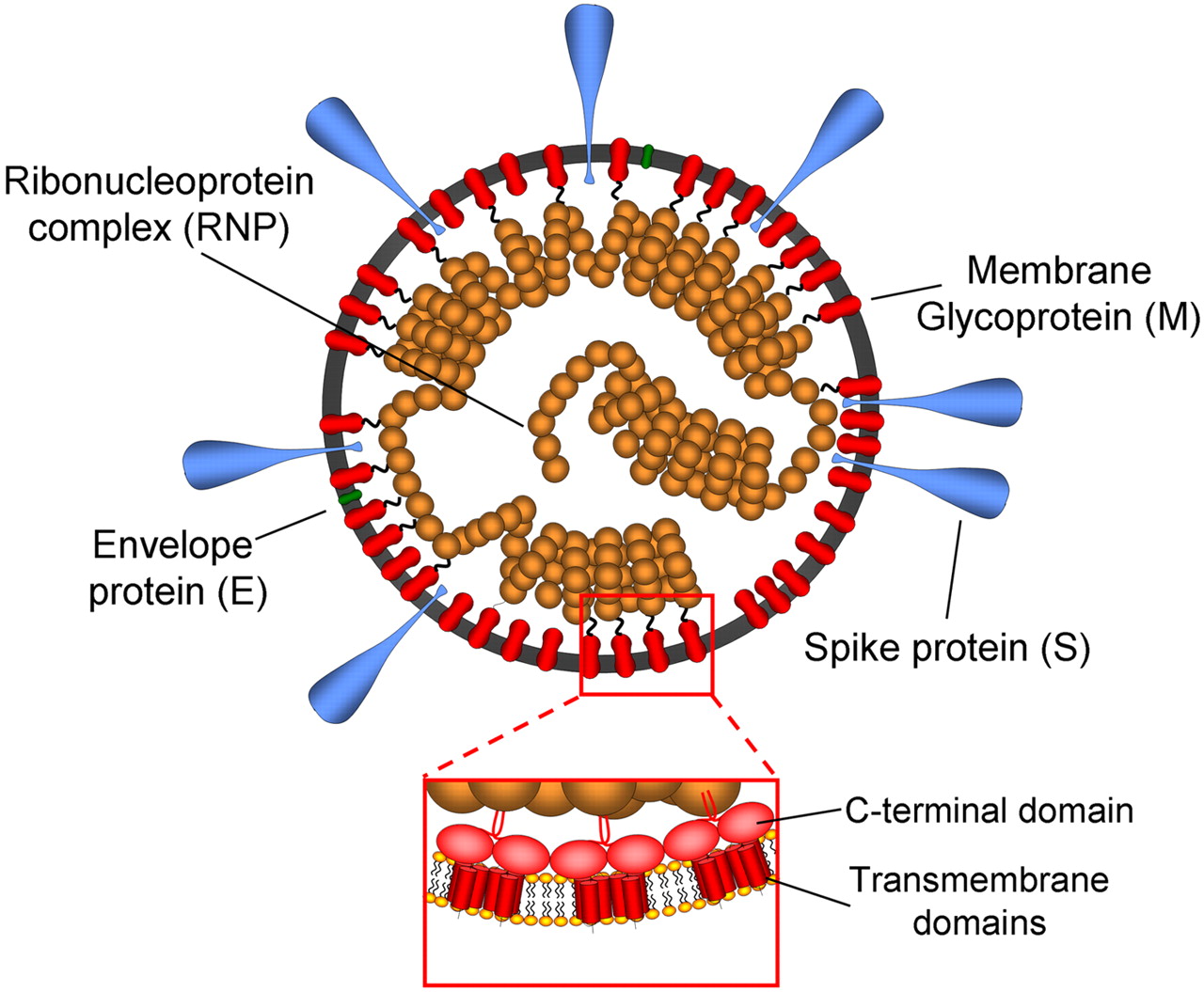
Yet if it weren’t for decades of basic science research on MHV, the vaccines that are our best hope for stemming the COVID-19 pandemic could not have been developed in record time. Like SARS-CoV-2, the viral culprit behind COVID-19, MHV is a coronavirus—a group that also includes the lethal pathogens responsible for outbreaks of Severe Acute Respiratory Syndrome (SARS) and of Middle East Respiratory Syndrome (MERS). Because of the family resemblance among these pathogens, says microbiologist Stanley Perlman, MD, PhD, of the University of Iowa, researchers’ investigations of MHV “set the stage for understanding the biology and pathogenesis” of its deadly cousins.
By delving into MHV, scientists were able to discover key clues as to how coronaviruses pick their host, invade its cells, reproduce, and cause illness. The arrival of SARS in 2002 and of MERS in 2012 provided opportunities to learn more about how coronaviruses spread and how they enter the human population. Such knowledge proved invaluable when SARS-CoV-2 emerged in 2019.
“There had been a lot of robust research devoted to understanding how these viruses work,” says Britt Glaunsinger, PhD, a virologist at the University of California, Berkeley. “That gave us a big head start when this new pandemic began.”
The Mouse Virus That Roared
Coronaviruses first came to light in the mid-1960s, thanks in part to a boy with the sniffles. Using samples of nasal fluid obtained from the child, virologist David Tyrrell, MD, of the U.K.’s Common Cold Research Unit and a colleague were able to detect the presence of a previously unknown virus. Later studies revealed that this virus belonged to the same family as MHV and viruses that trigger illness in pigs, cows, and other domesticated animals.
Because coronaviruses appeared to be responsible for nothing more serious than common colds in humans, they initially drew little scientific attention, UPenn’s Weiss says. Early in her career, she attended the first international conference on these viruses. Only about 60 people were there, she says, and “that was pretty much the whole [coronavirus] field.”
Weiss and Perlman joined this coterie for different reasons. Weiss became enthralled by MHV after reading a scientific paper about the virus, whereas Perlman, who began working on it in the early 1980s, was looking for insights into multiple sclerosis (MS). In this neurological disease, the insulation around nerves in the brain and spinal cord deteriorates, and a similar breakdown can occur when MHV infects a mouse’s brain. This overlap enabled Perlman and Weiss, who was also using the virus to investigate MS, to obtain grants from the National Multiple Sclerosis Society to support their work. Over the years, they also received funding from the National Institutes of Health.
A crucial discovery that researchers made while studying MHV would end up hastening the development of vaccines against SARS-CoV-2 more than 40 years later.
The scientists made a wise career choice. MHV, it turned out, was an ideal model for understanding how coronaviruses operate and trigger illness. One reason that MHV has been so valuable, says Weiss, is that it is much easier to study than its lethal relatives. The virus doesn’t infect humans, so scientists don’t need the complicated safety procedures and expensive protective equipment required to research SARS-CoV-2 and the viruses behind MERS and SARS, she points out.
Exposing Viral Dirty Tricks
A crucial discovery that researchers made while studying MHV would end up hastening the development of vaccines against SARS-CoV-2 more than 40 years later. Viruses exist to replicate, but they can’t copy themselves. Instead, they force a host cell to do the job. Before a coronavirus can turn a cell into a virus factory, however, it has to find a way to slip inside that cell. As researchers studying MHV learned in the 1970s, coronaviruses carry a molecular key now known as a spike protein, which provides access to individual cells in their human or animal host. Many copies of the spike protein protrude from the surface of each virus particle. When a coronavirus sidles up to a potential victim, the spike protein latches onto a receptor protein on the cell’s surface, and a series of molecular events then allows the virus to enter.
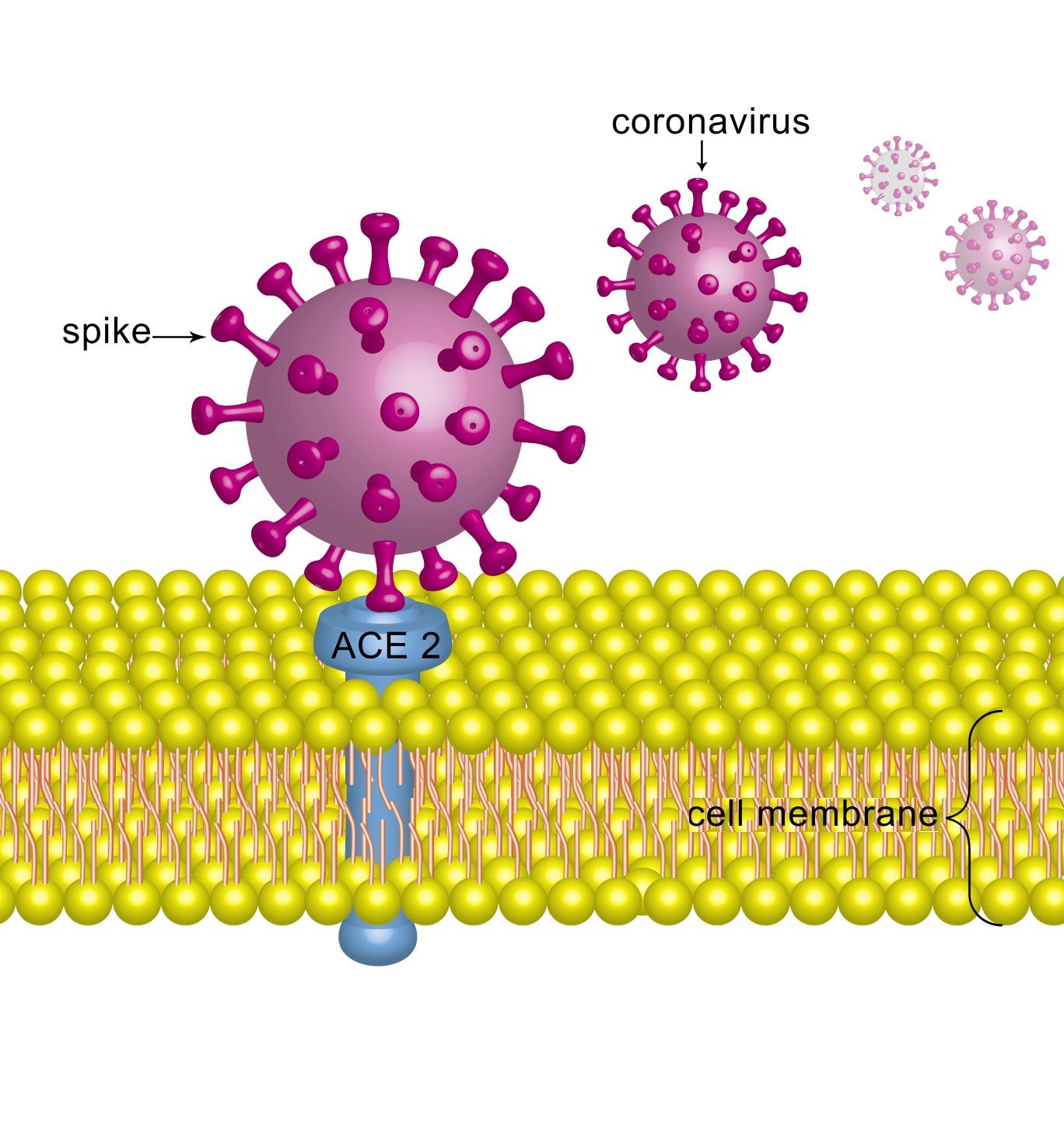
The spike protein is thus crucial for replication of the virus, so it makes a good target for vaccines. Indeed, most of the COVID-19 vaccines that have been approved or are under development are directed at this protein. These vaccines stimulate the body to produce defensive proteins called antibodies that hook onto the spike protein, preventing it from latching onto host cells’ receptors and blocking the virus from entering the cells.
The discovery of the spike protein was also significant because it helps determine which hosts—and which cells in those hosts—the virus can invade. Much as a key has to fit into a lock to open a door, the spike protein must match the shape of its target receptor before a coronavirus can gain entry to a cell. MHV fastens to a receptor protein called CEACAM1, which adorns a wide range of cells in a mouse’s body, including in the gastrointestinal and respiratory tracts. Human cells sport versions of CEACAM1 that differ from those of mice, explaining why MHV doesn’t infect our cells except under rare laboratory conditions.
Once inside a cell, MHV gets down to business. The virus contains a copy of its genome, the genetic instructions for making viral proteins. Unlike human cells, which store these instructions in DNA, MHV inscribes its genome in related type of molecule known as RNA. Using this blueprint as a guide, an infected cell obligingly begins manufacturing MHV proteins, providing the raw materials for a brood of new viruses. Many of these proteins, including the enzyme that makes new copies of the viral genome, perform similar roles across coronaviruses, and researchers were able to probe their functions by studying MHV.
A Self-Destructive Battle
MHV research has also provided important clues as to how coronaviruses interact with their hosts during an infection. The viruses are formidable foes, these studies revealed, because they are adroit at counteracting their host’s defenses. For instance, one way in which an infected mouse fights back against MHV is by deploying enzymes that chop up the viral genome. Weiss and her colleagues determined that MHV is able to short-circuit this response. They later discovered that MERS-CoV, the virus that causes MERS, performs a similar trick. Mice also try to protect themselves by releasing molecules called type I interferons that render cells more resistant to viruses, but MHV can thwart this countermeasure as well, Weiss and her team found. SARS-CoV-1, the virus that causes SARS, and SARS-CoV-2 also have this capability.
“Very few viruses kill us—we end up killing ourselves,” explains virologist Linfa Wang, PhD, of the Duke-NUS Medical School in Singapore.
By documenting MHV’s effects on mice, scientists have also gleaned insights into how coronaviruses induce illness and sometimes kill. Researchers investigating how the virus triggers the destruction of the insulation on nerves, such as occurs in multiple sclerosis, have discovered that the rodent’s immune system is actually responsible for much of the nerve damage. In studies partly funded by the National Multiple Sclerosis Society, Perlman and his colleagues found that certain immune system cells known as T cells, which were specialized to fight MHV, instead promoted destruction of the nerve insulation, suggesting that an overzealous response by the immune system underlies some of the health consequences of coronavirus infections.
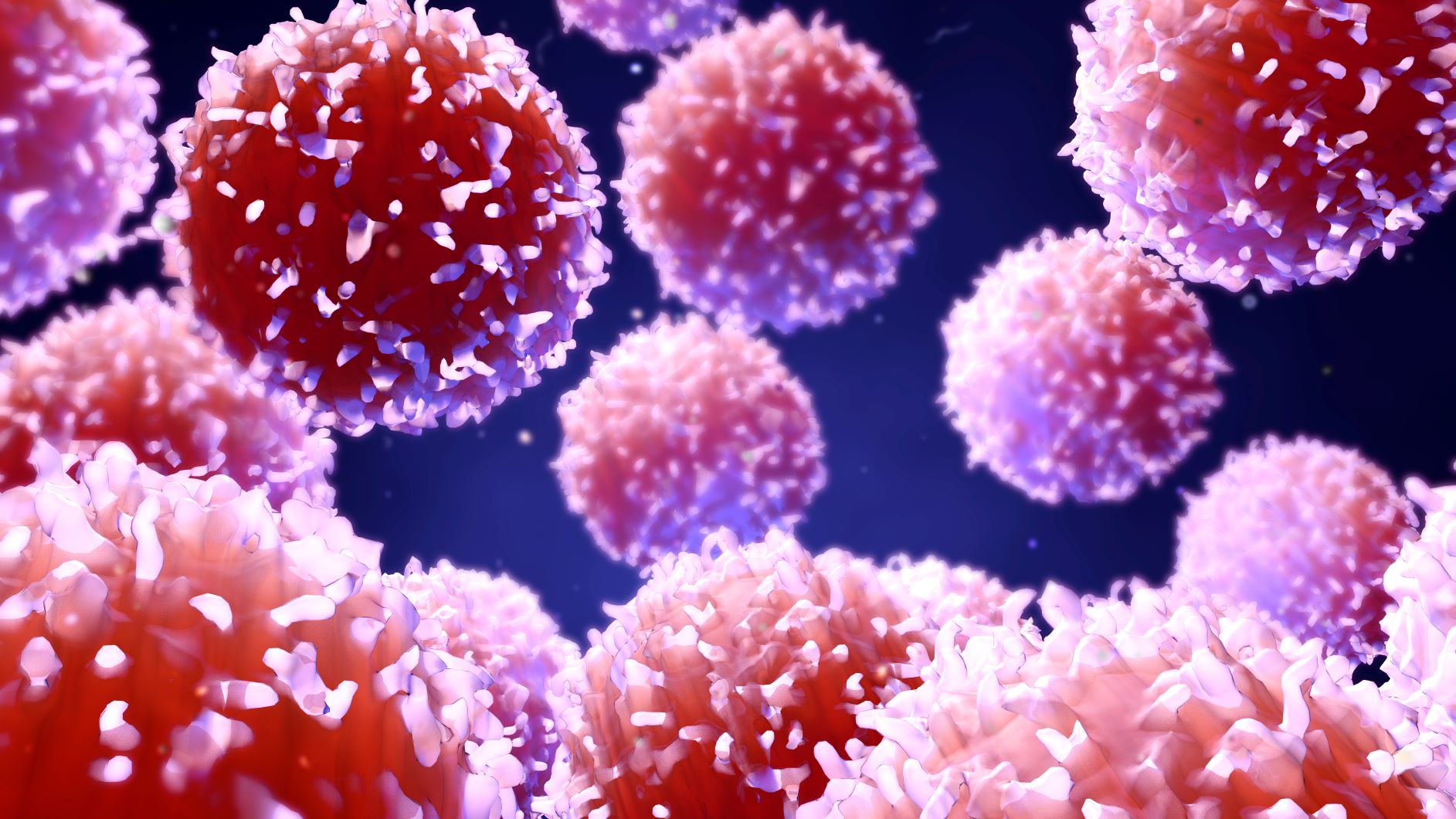
Scientists now suspect that the immune system overreacts in a similar way in many patients with SARS, MERS, and COVID-19—which could explain why these diseases are often lethal. When the immune system attacks the virus too aggressively, it may unleash rampant inflammation and other responses that trigger collateral damage in the lungs, heart, blood vessels, brain, and other parts of the body.
“Very few viruses kill us—we end up killing ourselves,” explains virologist Linfa Wang, PhD, of the Duke-NUS Medical School in Singapore. This self-destructive mechanism may also be one reason that coronavirus infections are more dangerous for older patients, he adds. As people age, the immune system doesn’t control its activity as tightly and becomes prone to an over-the-top response, Wang says.
Coronaviruses That Turn Deadly
Despite everything scientists had learned about coronaviruses from studying MHV, they weren’t prepared for what happened in 2002, when patients in Guangdong Province in China began falling ill from an unusual type of pneumonia. By the time the disease soon known as SARS mysteriously vanished, less than a year later, more than 8,000 people in 29 countries had become sick and nearly 800 had died. At the time, researchers knew of only two coronaviruses that infected people, both of which caused colds. When scientists zeroed in on a coronavirus as the culprit in SARS, “it was a total shock,” says Weiss.
About 75% of the infectious diseases that have recently begun affecting humans are zoonotic, or from animals, including AIDS, Ebola, Marburg hemorrhagic fever, Nipah virus infection, and West Nile fever.
But researchers soon discovered that SARS-CoV-1 was distinct in several ways from MHV and the other coronaviruses they were familiar with. Not only was it more dangerous, but the spike protein on SARS-CoV-1 also did not latch onto CEACAM1. Instead, it bound to ACE2, a receptor that juts out from cells throughout the body, including in the respiratory tract, kidneys, heart, and blood vessels. It turns out that ACE2 is also the docking site for SARS-CoV-2.
Tracking Coronaviruses To Their Surprising Source
The SARS outbreak sent researchers tramping through rain forests and poking into caves as they sought the origin of the new virus. Scientists suspected right away that SARS-CoV-1 had originated in an animal. Indeed, about 75% of the infectious diseases that have recently begun affecting humans are zoonotic, or from animals, including AIDS, Ebola, Marburg hemorrhagic fever, Nipah virus infection, and West Nile fever. Researchers initially homed in on the so-called wet markets in China, where live wild animals are sometimes sold for food. They found evidence of viruses similar to SARS-CoV-1 in three species of small mammals, including the cat-like masked palm civet, that were available in these markets. But researchers ruled out these three species as the initial source of the disease.
Wang, a member of the World Health Organization committee that investigated the origin of SARS, thought researchers should look in a different direction—up. He had studied the Nipah and Hendra viruses, bat-borne pathogens that had spread to humans in Australia and Asia, and he proposed searching in bats for the virus that spawned SARS-CoV-1. Some experts were skeptical about his plan. “They said, ‘Good luck,’” Wang recalls.
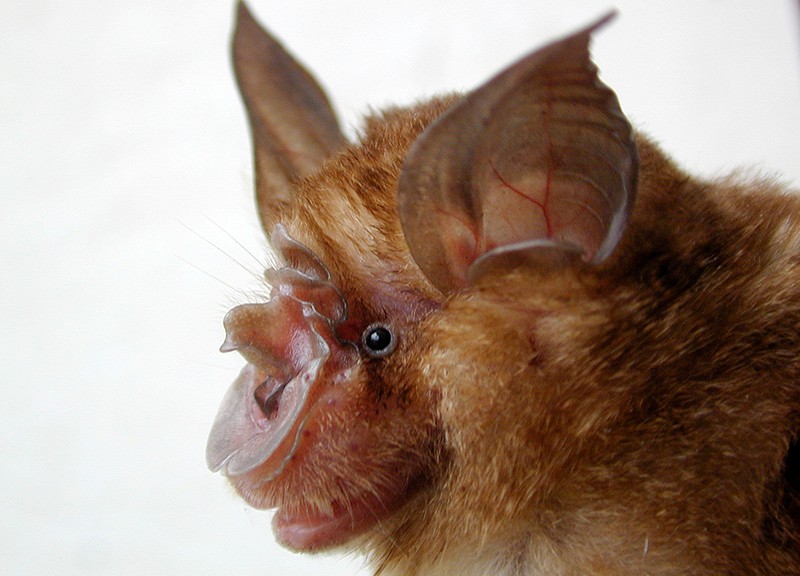
Undeterred, he joined forces with virologist Shi Zhengli, PhD, of the Wuhan Institute of Virology in China to pursue the idea. In 2005, Shi, Wang, and their team reported the discovery of viruses similar to SARS-CoV-1 in horseshoe bats captured in China. That same year, another group of researchers found kindred viruses in horseshoe bats dwelling in Hong Kong. And in a 2013 study of horseshoe bats in Yunnan Province in China, Shi and her colleagues detected viruses that are still closer genetically to SARS-CoV-1—providing the strongest evidence to date that the virus spread to humans from this group of bats.
Another Bat Virus Threatens The World
By then, the world had another new disease with a bat connection to worry about—MERS. Not long after the first cases turned up in Saudi Arabia and Jordan in 2012, researchers recognized that a coronavirus was responsible for this new illness, too. Between then and early 2021, MERS-CoV spread to 27 countries and infected about 2,500 people. The virus differs from SARS-CoV-1 in several ways. It is much more lethal, killing about 34% of its victims, versus fewer than 10% for SARS-CoV-1. And it remains a threat—62 people fell ill with the disease in 2020. MERS-CoV also diverges from SARS-CoV-1 and MHV in targeting a different receptor on cells, a protein known as DPP4.
“Bats are reservoirs of some of the most virulent disease-causing viruses,” says Cara Brook, PhD, a disease ecologist at the University of California, Berkeley.
Although humans can transmit MERS-CoV to each other, most people are likely infected by camels that harbor the virus. Camels, in turn, probably pick up the virus from bats. Studies conducted since 2012 have found viruses that resemble MERS-CoV in bats from Europe, Africa, and Asia.
Research on SARS and MERS has transformed our understanding of coronaviruses. We now know that these viruses can be deadly and that bats play a critical role in their evolution and spread. “Bats are reservoirs of some of the most virulent disease-causing viruses,” says Cara Brook, PhD, a disease ecologist at the University of California, Berkeley. And these flying mammals host plenty of coronaviruses.
“We predict that there are 3,500 coronaviruses infecting bats,” says Brook. She adds that bats may even serve as incubators for new coronaviruses, allowing the genomes of different viruses to mix and potentially produce more dangerous viral varieties.
Despite all of the valuable basic science research on coronaviruses conducted before the emergence of COVID-19, significant gaps in our knowledge remain. For example, although researchers warned that a new pandemic was likely and that an undiscovered coronavirus could cause it, they didn’t know where or when the event would begin—and thus they couldn’t forestall the explosion of COVID-19 around the globe. Studies by Shi and other scientists showed “it was easy for a virus to go from infecting a wild bat to causing human disease,” Brook says. “They raised the alarm, and still we allowed the pandemic to happen.”
The COVID-19 pandemic has underscored the value of basic science research by showing that it can yield practical benefits. “We didn’t start at zero” with coronaviruses, Glaunsinger adds.
To correct that failure, she and other researchers advocate a systematic international effort to monitor wild animal populations and identify viruses that might pose a threat to humans. The United States government sponsored one such program, known as PREDICT, from 2009 to 2019. However, the Trump administration shuttered the program just two months before the first COVID-19 cases were reported.
And despite all the early work on coronaviruses, we haven’t been able to develop treatments that can cure most patients. “We don’t yet have a miracle drug,” says Glaunsinger.
Nevertheless, the COVID-19 pandemic has underscored the value of basic science research by showing that it can yield practical benefits. “We didn’t start at zero” with coronaviruses, Glaunsinger adds.
We may need findings from basic science research that’s currently underway sooner than we’d like to admit, because researchers forecast that COVID-19 likely won’t be the last pandemic humanity faces this century. The world is teeming with viruses. Some day, another one will probably leap from an animal to humans and begin speeding around the globe. When that happens, seemingly esoteric findings from research on obscure viruses might be just what scientists need to craft vaccines and develop new treatments to fight the next pandemic.