Space is a cold and barren place. Nothing can exist there, nothing!” Ludwig Von Drake, an obscure uncle of Donald Duck and a professor of astronomy, is sitting on a high stool in his observatory. When he sees that he is being filmed, he falls off and lands on the floor with a loud thump. “Now I can see stars I’ve never seen before!” he groans. He walks over to a table with a large pile of books on it. The thickest of them all is a guide to space travel that he wrote himself. In a 45-minute-long monologue, he tells us in a thick German accent how mankind discovered the planets in our solar system and has fantasized about everything that might be crawling around on them. Every now and then, he picks up a book from the large pile and reads from it, and then throws it nonchalantly into a corner of the room. He tells us about Copernicus and Galileo, and about Kepler’s dreams about Martians, Fontenelle’s speculations about life on other planets, and even John Herschel’s Great Moon Hoax. Science fiction comes to life in the colorful cartoon: Hairy space beings and flying saucers shoot across the screen. At the end, the professor has the last word. He finds all these fantasies poppycock; nothing can live in that empty, barren space! But, as he is speaking, Von Drake is kidnapped by a black Martian robot from one of his stories.
The cartoon, Inside Outer Space, is part of Walt Disney’s Wonderful World of Color, a television series from the 1960s. The absentminded duck professor hosts a number of episodes, each with their own topic: the history of flight, the color spectrum, space—all exciting stuff for American kids in the Space Age.
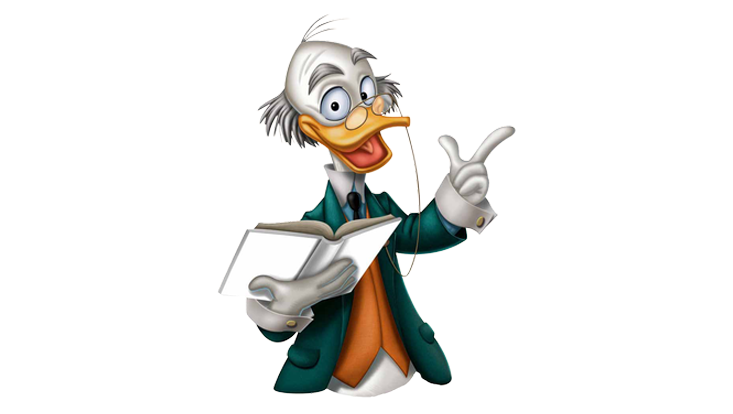
Lou Allamandola spent his teenage years in the science-crazy 1960s. He grew up in a Catholic family in the state of New Jersey. His grandparents were immigrants from Italy, and he didn’t learn to speak English until he went to school. He still clearly remembers the Disney cartoons with Ludwig Von Drake, which were broadcast on Saturday evenings. “Von Drake called the interstellar medium—the empty space between the stars and the planets—a barren place where nothing could exist,” he tells me. “That was all we knew in the ’60s. Now we know better. Interstellar space is full of molecules that we also see on Earth.”
I speak to Allamandola one Wednesday morning while he is visiting Leiden Observatory. He is a tall man with curly hair, greying at the temples. His accent reminds me a little of the Italian-American Mafiosi from The Sopranos. While we are talking, the door of his office opens now and again, colleagues who urgently need his opinion on their latest research results or to correct an article they are writing together. He tells them all to come back in the afternoon. “When I’m here, far away from my own office and telephone, I find it easier to say no,” he says. That office is at NASA’s Ames Research Center in California. Since 1983, Allamandola has been head of the Astrochemistry Laboratory, where they study how molecules behave in simulated space conditions. Astrochemistry—space chemistry—is a relatively new discipline, and Allamandola is a pioneer in the field.
On July 20, 1969, the peak of the Space Age, hundreds of millions of people sat glued to their televisions and radios, following Apollo 11’s moon landing. They heard Neil Armstrong’s words above the background interference: “That’s one small step for [a] man, one giant leap for mankind.”1
It is remarkable just how little we knew back then about the chemical composition of the interstellar space through which the astronauts were floating. Admittedly, compared to the Earth, space is very empty.
And yet, we knew that space wasn’t completely empty. At the beginning of the 20th century, telescope photos of areas with many stars showed strange dark spots where there were no stars at all. They proved to be large clouds of gas and cold space dust that absorb the light of the stars behind them. What lay hidden inside these dark clouds, however, could be made visible using spectroscopy.
Perhaps the chemical materials to create organisms on Earth originally came from space.
Every atom can absorb and emit at specific wavelengths, resulting in a fixed pattern of absorption and emission lines on the spectrum. This “fingerprint” can be measured with a spectrograph. Michel Mayor and Geoff Marcy measured the changes in wavelength of these lines in stellar spectra, so that they could use the Doppler method to determine the speed at which the stars were moving.
It is not only individual atoms that have spectral lines. Molecules—combinations of atoms—also emit light at certain wavelengths. These wavelengths are determined by the movements of the molecule. Hydrogen, the simplest molecule, comprises two hydrogen atoms that are joined together. That combination is possible because the two atoms share their two electrons. You can see it as two small balls connected by an elastic band (the electrons). Because the elastic is flexible, the atoms can move back and forth, like a kind of stretching exercise. And they can do that at varying speeds. If they change their speed or direction, they emit a light particle. These particles, called photons, each have a specific wavelength. That means that the light emitted by a gas cloud in space contains the spectral lines—the fingerprint—of the molecules within the cloud. In short, we can tell from the light from a gas cloud what kind of molecules it contains.
Molecules were not first detected in space until the mid 20th century. This had not been possible earlier because their spectral lines have very long wavelengths and can only be detected with radio and infrared telescopes. In 1800 William Herschel was the first to detect infrared radiation from space, but it would take a long time for better instruments to be developed.2
Radio astronomy, too, did not really pick up momentum until the 1960s, thanks to technology developed during World War II. Frank Drake and his companions used it for their early SETI experiments, but astronomers interested in star formation also studied radio waves. Gas and dust clouds were mainly found in the middle of groups of young stars, suggesting that stars were born in the clouds. As the cloud cools off, the particles within it move more slowly until the cloud collapses under its own gravity. The material in the middle of the cloud then condenses and a new star forms. Astronomers hoped to learn more about this process of formation by studying the radio spectral lines from the birth clouds.
The first molecules found in interstellar gas and dust clouds using radio observations had a very simple composition, with no more than two atoms per molecule.3 In March 1969 the discovery of the most complex molecule to date was announced: formaldehyde, which has the chemical formula CH2O. The article announcing the find, the lead author of which was radio astronomer Lewis Snyder, closed with the words that “molecules containing at least two atoms other than hydrogen can form in the interstellar medium.”
A certain degree of surprise can be detected in this statement: It had been assumed until then that there was nothing to be found in space. It was Ludwig Von Drake’s “barren place,” a godforsaken void where no molecule can survive. And now experiments were being conducted that suggested that the space between the stars was teeming with complex chemical matter. Snyder’s paper appeared four months before the moon landing, making the contrast even greater. Mankind could send astronauts into space, but had no idea of the chemical riches it contained.
Allamandola laughs and shakes his head when he thinks of all the many discoveries that were still awaiting astronomers. In 1968, he graduated in chemistry from St. Peter’s College, a small Catholic university in New Jersey. “Miraculously enough,” as he says himself, he was selected to conduct Ph.D. research at the prestigious Berkeley, which had one of the best chemistry departments in the country. His mentor was chemist George Pimentel, “a wonderful man, who possessed the skills of 10 people,” says Allamandola. One of the many interests of the multifaceted Pimentel, who also invented the chemical laser, was measuring the infrared spectra of gases in his laboratory. He wanted to apply this technology to find out whether there was life on Mars by detecting gases originating from life forms. NASA sent a spectrograph he had built himself on the unmanned Mariner spacecraft that flew past the red planet. The spectrograph did not discover any biological material, but did provide a lot of information on the temperature and conditions on the planet’s surface. Following this, NASA selected Pimentel to be part of the first group of scientists to be trained as astronauts. He withdrew from the program, however, when it became clear that he would probably never go into space.
While studying under Pimentel, Lou Allamandola learned about infrared spectroscopy in the lab. After getting his Ph.D., he found a research position in Oregon, a little farther up the west coast of the U.S. When his contract expired in 1976, it was difficult to find a new job. “The oil crisis had hit, and there was little money available for research,” he explains. “Instead of the four or five offers I would normally have got 10 years previously, I had about 80 rejections. My wife and I had just had our second child and we were a little at a loss about our future. Then I had a call from George Pimentel. He had heard about a position that was made for me. An acquaintance of his, theoretical astronomer Mayo Greenberg, wanted to set up a lab to simulate chemical processes in interstellar dust clouds. That was music to my ears. Then George said ‘Just one snag. How is your Dutch?’ ”
In the vicinity of stars, the ice mantle of the space dust grains became molecule factories, in which a wide range of complex structures could be produced.
During his subsequent telephone calls with Greenberg, Allamandola became more and more enthusiastic about the work he would be doing in Greenberg’s lab in Leiden. Until then, astronomers had mainly found space dust irritating, as dark dust clouds obstructed their view of areas where stars were being formed. But Greenberg found them fascinating. He suspected that grains of space dust were covered in an outer layer of water ice, like snowballs, in which other chemicals—for example oxygen and carbon—were dissolved. Allamandola explains how Greenberg came to this conclusion: “Space dust contains the element silicon, just like glass. Water vapor drifting around in space condenses onto the silicon in the same way that, here on Earth, we see ice flowers on our windows when it freezes outside. The glass cools the air and the vapor in the air freezes. It ain’t magic, but for some reason or another, the snowballs had not yet occurred to most astronomers.”
Greenberg and Allamandola became interested in the frozen dust grains because all kinds of chemical processes could occur in them that were impossible elsewhere in space. “Imagine a lonesome molecule drifting through the vacuum of space,” Allamandola explains. “After a few hundred million years, it comes across another molecule with which it reacts and forms a new molecule. That process will be speeded up if the molecules are more closely packed together in ice that has settled on space dust.”
The ice, which—compared to interstellar space—has a very high density, acts as a kind of meeting place for molecules. When the surface of a dust grain is illuminated by a star, it activates all kinds of chemical processes. The energy supplied by the ultraviolet starlight enables larger molecules to be formed from small chemical building blocks.4 If Greenberg’s suspicions were to prove correct, a wide range of complex molecules could be formed in the interstellar ice grains. Perhaps the chemical materials to create organisms on Earth originally came from space.
And so, in 1976, Allamandola and his young family moved to Leiden. He was to stay there for eight years, and he says his Dutch is “still reasonable.” He shows me a picture of the research team at the Leiden laboratory in 1970s. Eight men and one woman. They have long hair, black-rimmed glasses, some of the men have thick beards. Greenberg himself is in front of the group: a small man with gray hair, a blue rollneck sweater and a tweed jacket. The laboratory assistants are surrounded by complex-looking equipment.
Allamandola tells me that doing research in the 1970s was very different from now. “We didn’t have these things,” he says, tapping his laptop screen. “It used to be normal to talk to each other for hours in the canteen. About science. If you wanted to read an article, you went to the library, where you could spend the afternoon thinking in complete peace and quiet. I don’t know many people now who still spend an afternoon sitting and reading. There is always the pressure to do so many other things. At conferences, people check their emails rather than listen to the speaker. On your laptop, you have the entire canon of scientific literature at your fingertips, but that doesn’t mean you absorb the information more quickly. There are Schwarzenegger movies about how machines will take over the world. In my opinion, in a certain way, they already have.”
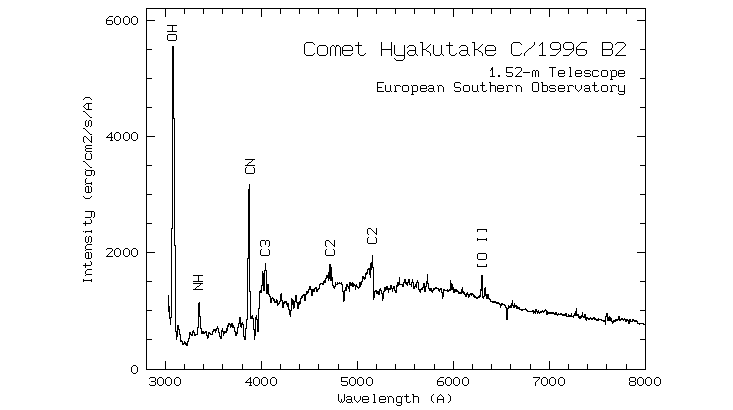
Allamandola shows me the next photograph, a closeup of the machine that the researchers were standing around. “Look, this is an ice simulation chamber. Normally, I don’t like having to explain complicated measuring equipment, but this is so simple. It exactly recreates the situation in space that we want to replicate.” Without an explanation, the machine indeed looks complicated, a little like the inside of a computer. There is a lamp aimed at a kind of biscuit tin with a tube screwed to it. “This emitted ultraviolet light and simulated the star,” Allamandola says, pointing to the lamp. “The biscuit tin represented the dust cloud; inside it was a deeply cooled sample of water ice containing ammonia and carbon monoxide, two common molecules in space. The tube behind it was the spectrograph. That captured the light, registered whether molecules had been formed in the ice and, if so, which ones.”
It worked. Allamandola shows me two spectra—one before and the other two hours after radiation with ultraviolet light. The first spectrum showed only lines of water, carbon monoxide, and ammonia—the ingredients of the ice sample. But the second contained a large number of new spectral lines, indicating the presence of new, larger molecules that had been formed from the basic ingredients.
This result was spectacular. In the vicinity of stars, the ice mantle of the space dust grains became molecule factories, in which a wide range of complex structures could be produced. In 1969, scientists had been surprised to discover that a complicated molecule like formaldehyde could be formed in space. Yet in the ice chambers in Leiden, under the same conditions as in space, it was produced in great quantities from the 1970s onward.
But the results of the laboratory experiments were not immediately noticed and accepted by others. “Astrochemistry was still a young discipline,” Allamandola tells me. “Scientists discovered more and more new molecules in space. They constructed theoretical models that show how the molecules—in gas form, not in an ice crystal—could have formed. The fact that these reactions could never take place if the molecules were just drifting separately through space was ignored. Astrochemists were just fine without our icy grains. They saw us as nutty professors.”
That all changed in the 1980s when Allamandola and his colleagues, including Leiden astronomer Xander Tielens, conducted observations on board the Kuiper Airborne Observatory—a converted Lockheed aircraft fitted with a telescope and spectrograph. The telescope was behind a hatch in the side of the fuselage. An airlock made sure that the researchers were not sucked out of the plane as a result of the fall in cabin pressure when the hatch was opened. Because the plane could climb above the water vapor layer in the atmosphere, it was possible to measure the water vapor and ice in space. And they found the ice grains: Dust clouds from which stars and planets were formed contained water ice and the same complex molecules that had been produced in the laboratories in Leiden and Ames.
During a conference in Australia in 2010, I myself first heard about the many molecules that have been found in interstellar space since that time. The conference dinner was on Magnetic Island, off the east coast of Queensland. On the restaurant lawn, possums scratched around between the laid-out tables. Some 200 astronomers had just finished their dessert and Andrew Walsh, the conference organizer, was speaking. Walsh is a slightly curt Australian with little hair on his head and a beard in two impressive long plaits. Besides astronomy, his great love is brewing beer.
Space is not the barren, empty place that Ludwig Von Drake described, but is teeming with the building blocks of organic life.
“My father asked me when I started doing my Ph.D. in astronomy ‘So what do you actually spend your days doing?’ ” Walsh told us. “I told him the title of my dissertation: ‘The Association of Ultracompact HII Regions and Methanol Maser Emission.’ He looked at me glassy-eyed, and I could see that I was losing his attention—until I said the word ‘methanol.’ ‘Aha!’ he said, ‘so you have alcohol in space? Is there beer in space?’ I explained to him that ethanol, not methanol, is the alcohol you get in beer. ‘Methanol is a poison, Dad,’ I said. ‘If you drink just a little of it, you’ll go blind. If you drink any more, you’ll die.’ From that moment, my father lost all interest in my work. I’d like to put that right with this presentation, which I give the title ‘Beer in Space’ and dedicate to my father.”
In 15 minutes, Walsh—who became more and more animated—listed the 12 main ingredients of beer. Water, alcohol (ethanol), sugars, a few amino acids. Then he showed us photographs of the areas where stars are formed—the same dust clouds that Allamandola simulated with his laboratory ice. Enthusiastically, one after the other, Walsh named the ingredients of beer that have been found in all these clouds: plenty of water and ethanol, carbon dioxide, even sugars and a few simple amino acids. Five of the more complex amino acids and sugars have not yet been found, but Walsh is convinced that that is because we haven’t looked hard enough yet. He called on his colleagues to keep looking for the missing ingredients for the space beer. “It would be very reassuring for my father and many others to hear that we also find useful things in space,” he concludes.
From the 1980s, astronomers had not only found some of the ingredients for beer in space, but had started a tentative search for the basic materials of life. Lou Allamandola returned to the U.S. in 1983, where he set up his own laboratory at Ames to continue the experiments he had conducted in Leiden. “The list of substances we made in the lab got so long that even chemists were starting to find it boring. At the end of the ’80s, we wanted to see whether we could also make molecules that resembled the building blocks of living organisms.” I ask Allamandola whether, as a religious man, it is difficult to combine his faith with a study of the origins of life. “Not at all,” he says. “Religion and science are different domains—and both contain great mysteries. Besides, the chemistry I study is still so far removed from the origins of life.”
Some of the experiments conducted by the Allamandola team produced remarkable results. After each experiment, the radiated ice was thawed out and dissolved in water. The liquid was then heated up so that the water evaporated. What was left was an oily residue that Mayo Greenberg had already christened “the yellow stuff” in his early experiments. Perhaps there was something in the yellow stuff that was too complex to have been picked up earlier by the spectroscope? Greenberg made headlines in the Netherlands in 1980, when he suspected that the residue also contained amino acids. Amino acids are the basis of proteins in our bodies and are the building blocks of life. “Origin of Cosmic Life Simulated in the Lab” was perhaps the least dramatic headline. The local Leidse Courant had no such reservations, stating with wild exaggeration “Leiden Researchers Find Life Among the Stars.”
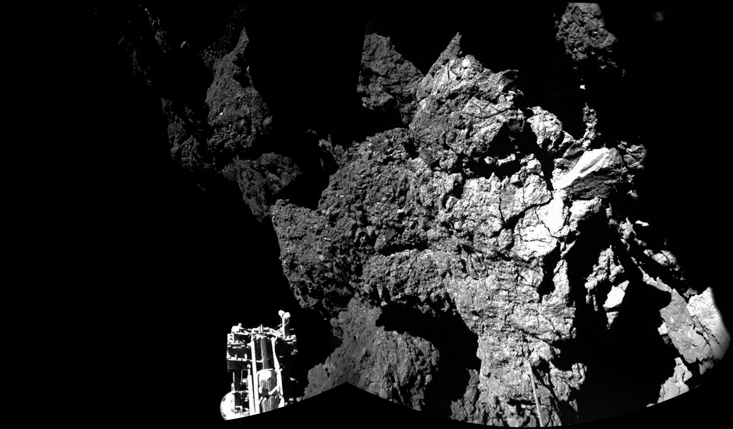
“Of course we hadn’t made living organisms,” says Allamandola. “You always have to watch what you say, otherwise people get the wrong idea. Prebiotic, biogenic … in other words, the same building blocks from which life is made. A human being, even a living cell, is an enormously complex Lego construction. All we found were a few individual Lego bricks, not the whole structure.” But they did find an enormous variety of chemical building blocks under the microscope. Besides amino acids, there were also sugars and even nucleic acids, which form the basis of DNA. They also found elongated molecules that repelled water on one side (hydrophobic) and bond easily with water on the other (hydrophylic). The cell membranes of the human body are made of the same type of molecules.
As Allamandola tells me all this, I become as enthusiastic as the journalist from the Leidse Courant. They’d discovered that life in space is possible! Allamandola spreads his arms and gestures me to calm down. “Ho ho, Lucas,” he says. “No one knows what life is. There are about 500 different definitions. What we have found has nothing to do with life, as yet. All we have found is the building blocks; how they eventually lead to a living organism is a completely different matter.”
Scientists have been addressing this question for hundreds of years. In the 1950s Miller and Urey conducted experiments to explore Darwin’s idea that life on Earth had been formed in a warm little pond that was struck by lightning. Complex molecules like amino acids were produced in their test environment, which was later more or less replicated by Bill Borucki. The experiments by Allamandola and Greenberg showed that the same substances could be created in a block of ice in space that had been radiated by a star. The big question was, how did these substances end up on Earth?
The Earth most likely originated as a hot ball of liquid rock. Some 4 billion years ago, it had sufficiently cooled for life to evolve. The oldest fossils found on Earth are of bacteria that developed around that time. The ice experiments showed that we could also find the basic materials for these organisms in space. Could these molecules, via a sort of cosmic postal service, have been delivered to the Earth after it cooled off? Panspermia, the hypothesis that life on Earth originated in space, was starting to look like an interesting possibility.
In 1989 Allamandola met biochemist David Deamer. At that time, Deamer had a fragment from a meteorite that had impacted in Australia. An enormous chunk of rock weighing around 100 kilograms had broken up into smaller pieces in the atmosphere. The fragments were later analyzed in a laboratory. Deamer’s meteorite proved to have the same cell-wall-like structure that Allamandola had created in his lab. It was a remarkable find, showing that meteorites that impact the Earth contain the basic materials for organisms. The time was not yet right, however, to draw far-reaching conclusions. “There are still people who leave the room if they hear the word biomarker—an indicator of life—in a talk. I simply did not dare to show some of our results, which suggested that the building blocks of life can be formed in meteorites. If I were to do that, whether it was at a chemistry or an astronomy conference, my colleagues would think I had gone insane.”
In the mid 1990s, however, astrobiology became increasingly popular. In 1996, Allamandola was the speaker at a workshop organized by NASA and SETI on the island of Capri, off the west coast of Italy. At the end of his presentation, he dared show a slide depicting the structures of Deamer’s meteorite alongside those from his own laboratory. “The time was ripe,” he tells me. “People were open to the idea that organic materials on Earth may have been delivered by meteorites.”
Since then, there has been a growing awareness that many of the substances that we absorb on a daily basis were formed in space. Take water, for example. Every meteorite or comet is an enormous snowball with its origins in the birth cloud of the solar system. If such an object impacts the Earth, it deposits a large quantity of water onto the planet’s surface. It is difficult to imagine that enough of these snowballs have fallen to Earth to create all the oceans, but I recently saw an image that made this idea a little more acceptable. It was a drawing of a dried-up Earth, with the water from all the rivers, oceans, lakes and so on condensed into three small spheres. The largest of the spheres—in diameter about the same as the distance from Amsterdam to Rome—represents all the water in, on or above the Earth. It is pretty small compared to the size of the Earth. Suddenly, it didn’t seem so strange after all that every glass of water, every cup of tea and every beer that I had ever drunk had once been part of a snowball in space.
A meteorite impact may not seem like something that happens every day, but it is. Only the larger impacts make the news, but thousands of kilograms of interstellar material land on the Earth daily, in the form of small meteorites and space dust. In the young solar system, the impacts were even more frequent and violent. Dating of craters on the moon shows that around 4 billion years ago, enormous showers of meteors passed through the solar system for a period of millions of years. These must have impacted on the Earth as well as the moon.
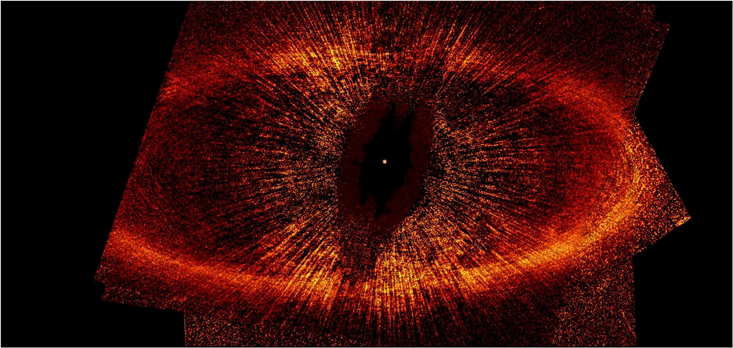
One possible explanation for these meteorite showers is that, shortly after it was formed, the planet Jupiter moved a little closer to the sun. This migration was apparently caused by the gravity of other planets and small objects orbiting the sun. The shift in Jupiter’s orbit must have knocked the rest of the solar system out of balance and acted as a sort of catapult on the space rubble flying around the planets. Consequently, the inner planets—including the Earth—were severely bombarded with meteorites for a long period. This event has come to be known as the Late Heavy Bombardment. Similar bombardments are also observed today around young stars that are still in the process of formation. Space dust and water are thrown around by the embryonic planetary system and end up on the planets as they cool off.
One of the most well known images from the Hubble Space Telescope has been nicknamed “The Eye of Sauron” by astronomers, because of its close resemblance to the symbol of the Dark Lord in the Lord of the Rings movies. The image shows a kind of golden halo, surrounded by an oval ring. In the center of the ring, the star has been removed because it is too bright. This has left an elongated dark spot on the image that looks like the pupil of an eye.
The image is of Fomalhaut, one of the closest stars to the Earth. The oval is reflected light from a ring of space dust. The dust is probably produced by comets and other space rubble flying through the system at random. Every day, thousands of objects collide, break into smaller pieces and produce space dust that is full of water and organic molecules. Large and smaller fragments eventually land on the young planets orbiting the young star. The comet shower in Fomalhaut shows us what the Late Heavy Bombardment probably looked like.
We are currently finding out a lot more about these water-carrying projectiles in our own solar system. In 2014, the spacecraft Rosetta reached comet 67P/ChuryumovGerasimenko. It sent down the lander Philae, while the mother ship continued to orbit the comet for two years before crashing intentionally on its surface. Rosetta and Philae found water, oxygen, and numerous organic compounds (not to be confused with living organisms) in the comet. Curiously, the molecular makeup of the comet’s water was very different from the water on Earth, suggesting that comets—or at least, comets like 67P—might not have contributed greatly to the delivery of water to Earth. The eventful Rosetta mission marked the first time in history that comet water and dust could be studied directly.
When I had finished talking to Allamandola, I felt as though I myself had undertaken a cosmic journey. In the two hours we spent together in his office in Leiden, we followed the passage of an organic molecule through space; from its formation in a frozen grain of dust in the birth cloud of a young star, via the disc of dust and gas in which planets are formed, to its arrival on a planet through a meteorite impact.
It is a route that is still the subject of intense study by astronomers, including those in the Netherlands. Allamandola is in Leiden to give lectures at the two leading astrochemical research groups located there, one of which is led by his friend and former colleague Xander Tielens. Telescopes like the infrared satellite Herschel and ALMA, an array of several dozen radio dishes in the Chilean Andes, are exposing parts of the spectrum that were previously not accessible. This produces new spectral lines and new molecules in star formation regions.
These observations make some planet hunters optimistic about the chances of life existing on exoplanets. After all, the materials from which the inhabitants of Earth are made are also found in young planetary systems. Space is not the barren, empty place that Ludwig Von Drake described, but is teeming with the building blocks of organic life. Dissolved in water, these materials are continually delivered to the surface of young planets by meteorites. If the temperature is right and the ingredients are present, time and evolution do the rest. Perhaps it was this line of thought that led planet hunter Steven Vogt to make his “100 percent” claim about Zarmina’s World.
Yet just how the route runs from building blocks, via chemical reactions, to life remains unclear. We do not even know how it happened on Earth. Direct evidence—for example, the earliest life forms—have as far as we know largely disappeared from the face of the Earth. There are too many uncertainties to give preference to any single theory on the origins of life above all the others. And, for that reason, we cannot use life on Earth as a blueprint for the rest of the universe. Most planet hunters take a different approach to the question of whether extraterrestrial life exists. Imagine that a certain life form had developed on another planet from the same building blocks that we use on Earth and that we see everywhere in space. How then could we detect the existence of that life form from the Earth? How can we recognize a sign of life from an exoplanet?
Lucas Ellerbroek is an astronomer and researcher in comets and planet formation at the University of Amsterdam.
Reprinted with permission from Planet Hunters: The Search for Extraterrestial Life by Lucas Ellerbroek, published by Reaktion Books Ltd. © 2017 Lucas Ellerbroek. All rights reserved.
Exclusive to Nautilus readers: Buy a copy of Planet Hunters at 20 percent off the retail price of $27 with promo code PRHUNT20.
Photocollage credits: Mariyana M / Pavelis / Shutterstock