After midnight in a sweltering room in Pasadena in July 1976, Viking Mars team members sat hunched around a bulky monotone computer monitor, tensely awaiting the first data from the world’s first successful Mars probe lander, the only Mars lander ever specifically designed to detect life. Over the next weeks each of Viking’s first life-detection experiments came back with a striking signature. As the data trickled back into the Space Operations Facility, it became clear that carbon dioxide was released when organic compounds were added to Martian soil, though not when the mixture was superheated. This was a life signature, and exactly what had happened with the experiment on Earth. When water was added to the soil, oxygen was released, just as on Earth. The remote probe, panning for life, had found its signature in its first two experiments. The third experiment heated the soil, like warming food in the oven, and those results were mixed.
Arguments intensified, however, as the fourth experiment’s conflicting data came in. To claim life on Mars would be unprecedented. If they were wrong, no team member would live it down. Anything was better than striking out with a pompous grin on your face. Unbeknownst to most in the world watching, however, three of the four experiments on the primitive Viking lander could have been interpreted as testing positive for microbes, giving the same results as when they had been checked thousands of times on Earth. The researcher Patricia Straat told another mission staffer, Gil Levin, “That’s life!”
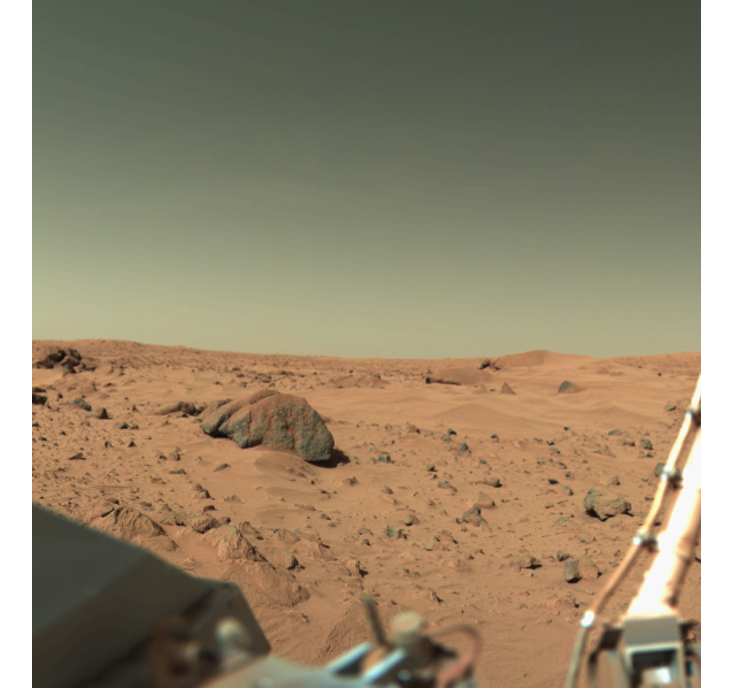
However, the fourth experiment, employing a gas chromatograph of the kind employed by James Lovelock, and a mass spectrometer, a delicate instrument for measuring the size of molecules, showed no life—and not only that, but absolutely no organics—on Mars. That was a stunning result: Organics exist all over space—on asteroids, comets, and meteors, and in interstellar dust. Not only that, but the experiment suggested that Mars’s surface was poisonous or self-sterilizing. Mission scientists had a heated argument, and NASA eventually decided to err on the side of caution. The surface must be self-sterilizing, they concluded, owing to the planet soil’s powerful oxidizing agents, which also helped to give it its red color. Viking had found a barren, windblown red planet, pockmarked by craters, cold and dead as the moon.
A few mission scientists disagreed, maintaining that the fourth experiment simply failed—as it had often in Earth tests. A group of activists, including Levin, wrote and spoke, goading NASA to release the full Viking data. On NASA’s 2016 celebration of the mission’s 40th anniversary, he repeated his call. He predicted the Mars Curiosity rover would find complex organics, which it did. When he saw the detection of methane bursts by Curiosity, he told me, he saw that the disappearance of the methane had happened too rapidly to have been caused by ultraviolet radiation: “This disappearance could have been caused by methanotrophs, which use methane and makes for a perfect little eco-cycle.”
Other Mars probes gave conflicting results. NASA’s Opportunity and Spirit rovers in the early 2000s, whose reports thrilled millions of fans around the world, including me, were designed and constructed by geologists and engineers, not by biologists. Evidence of water came from the 2008 Phoenix lander, whose camera pictured clear droplets on its cold steel legs. Simulations suggested that either water condensed around windblown grains of calcium perchlorate, the salt-type mineral whose properties enable it to scavenge water from the atmosphere, or that the landing had stirred up dirty ice beneath the surface and drops had formed and melted on the legs. The point was, said the University of Michigan’s mission scientist Nilton Renno, “On Earth, everywhere there’s liquid water, there is microbial life.” Such saline water on Earth housed microbes, in fact.
Suddenly everyone was interested in what could live in an ice-covered lake or plain, or in a remote cave or mine miles below the planet’s surface.
One of the best places to look for promising bits of Mars, paradoxically, was Earth. Walk along the frozen white expanse of the Antarctic and you will see bits of Mars, in the form of small stones. In fact, about 10 pounds’ worth of rock from Mars falls on Earth every year. If a large meteorite strikes Mars, it flings bits of rock into space beyond the small planet’s gravity. As that planet’s closest neighbor, our own, larger planet will find that its gravity traps some of the rocks, which fall to Earth’s surface and are most easily found in barren, ice-covered regions such as the Antarctic. Their authenticity is determined by chemical analysis of their shock glass, the melted glassy substance original to the rock. If a stone’s shock glass contains the exactly the same mix of gases as Mars’s atmosphere, identified in the many Martian probes, it is from Mars.
Tiny squiggles on a famous Martian meteorite found in Antarctica, ALH 84001, in 1996 led the NASA researcher Dave McKay and his team to claim that they had discovered fossils of microbes. Today most regard that as unlikely. But eons ago abundant water had certainly flowed in Mars’s oceans and rivers, the liquid’s mineralized remnants clearly visible across the planet—floodplains, alluvial basins, even oxbow curves in long-dried great rivers. The original name given in 1887 by the astronomer Luigi Schiaparelli to the great rift valleys seen through early telescopes was canali, Italian for “channels” (though English-speaking researchers translated it incorrectly as “canals”). At the turn of the century, in Arizona, Percival Lowell thought he glimpsed active Mars rivers featuring seasonal changes in vegetation. In fact, numerous probes have imaged morning haze in Martian canyons. Seizing on Lowell’s claim, Edgar Rice Burroughs, the author of the Tarzan books, wrote a wacky series of 1920s and ’30s Princess of Mars science-fiction novels that sent generations of young Americans to adventure. What Lowell saw was flaws in his mirrors. What Burroughs saw, as he divorced his wife for a Hollywood actress, was a gold mine of public gullibility.
Later NASA probes produced confounding results. The Mariner probes in the 1960s strongly suggested that Mars’s thin, cold atmosphere permitted no possibility of pure liquid water, though the final orbiter clearly pictured the beds of ancient streams and oceans.
In 2010 a University of Arizona undergraduate studying the Mars orbiter images spotted intermittent dark streaks running in parallel at ridgetops, then disappearing, as if in seasonal flow. What Lujendra Ojha noticed in the puzzling phenomena came from the high-resolution imaging science experiment (HiRISE). The seeping dark streamlets appeared at dozens of sites. Excited, Ojha paired HiRISE observations with mineral maps of Mars. The spectrometer observations showed hydrated salts at several locations, but only when the dark features appeared and widened. Using the orbiter’s compact reconnaissance imaging spectrometers, Ojha and the team then analyzed light reflecting off the streaks, detecting in it traces of either sodium perchlorate or magnesium perchlorate. Mars water contained a natural briny antifreeze.
Picture a cool planet with intermittent water and dryness and the largest volcano in the solar system. Giant lakes contain the same volume of water as that in the Arctic Ocean, fed by rushing rivers that deposit tons of alluvial sediment in their deltas. That was early Mars. Now picture a brimstone-smelling, acidic, ocean-covered planet with a toxic atmosphere and hot greenhouse gases, with neither oxygen nor radiation-sheltering ozone, that was pummeled by comets, then rammed by a Mars-sized planet, ejecting enough rock to form a gigantic moon that wrenched the planet’s surface into skyscraper-high tides. Welcome to early Earth.
For that and other reasons, the NASA investigator Steven Benner and others suggested that life originated on Mars and was carried to Earth by ejecta. Poring over the old Viking transcripts in the Houston NASA library, Benner found precious clues in the 40-year-old transcript to what they had seen in the hot nights as the data unfolded. What he found was “mass confusion,” he told me. Studying the DNA of ancient microbes and resurrecting their genes and proteins, Benner sought to connect the origin of life on Earth with the existence of life in the solar system. Mars, he pointed out in a series of papers, featured “warm temperatures and a wet dry cycle,” he said, enabling RNA building blocks to concentrate “for our kind of chemistry.”
The trouble was that many past claims of Martian life or water had been so wrong. But many ancient microbes had thrived in similar icy, alkaline environments on Earth. For that reason researchers raced to sulfurous caves, hot springs in Kamchatka containing molybdenum and borate, Yellowstone National Park, and the brine lakes of the Ant- arctic. What they found was beguiling.
NASA’s Chris McKay and Penelope Boston were two of the researchers searching remote, extreme outposts on Earth for signs of microbe metabolism and origins. A former New Mexico School Institute of Mining and Technology professor and the daughter of two circus trainers, Boston started off studying microbes in the Arctic and then switched to searching in deep caves. California’s Alison Murray sought extreme microbes in the Antarctic. Suddenly, after Curiosity, everyone was interested in what could live in an ice-covered lake or plain, or in a remote cave or mine miles below the planet’s surface. The likelihood of microbial life on Mars, which Boston had placed at 30 percent, was now, in her view, increasing. If tiny forms of life exist in hostile lake, cave, or mine environments, Boston reasoned, microbial life could eke out an existence on the subsurfaces of Mars.
Starting as director of the Cave and Karst Studies Program at the Institute and cofounder of New Mexico’s National Cave and Karst Research Institute, Boston made the case for Mars, helping to create the Mars Underground and putting together a series of discussions in which she started to win over the skeptics at NASA for life on Mars as a significant possibility. She was so successful that in 2016 the space agency named her the new director of its Astrobiology Institute in Moffett Field, California, giving her the exciting opportunity to “help guide the science I love at a very high level,” she told me.
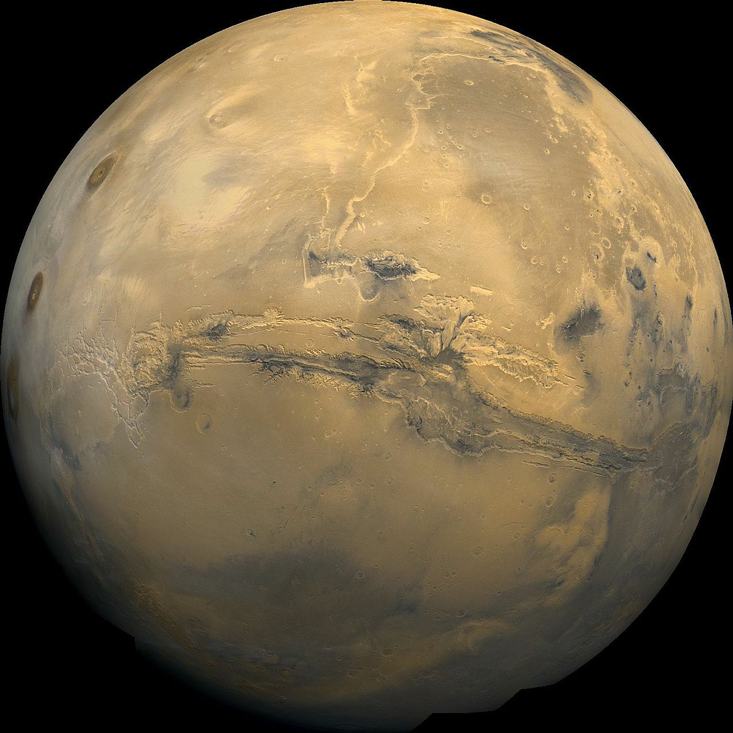
From Nevada, the Desert Research Institute biochemist Alison Murray joined Louisiana State University geophysicist Peter Doran to study the microbes and ancient climates of the briny, ice-covered lakes of the Antarctic, together finding a wide variety of Archaea and bacteria. “They don’t do much—they hibernate most of the time,” said Murray, who drilled ice cores from the Antarctic Lake Vida, “but they’re there.” It was warmer further down below the brine, but the core tubes brought up ice from the deep sink.
That insight took researchers back to the American west, where the Berkeley biologist Jill Banfield studied the Colorado River and the water of an abandoned California mine at Iron Mountain. Banfield discovered several new phyla of bacteria in a single toxic mine-waste site. The key was that these strange, previously unknown microbes depended on communities of other organisms to survive. This helped explain why so few could be grown in the lab. Working in a shallow aquifer near the Colorado River, Banfield’s team applied a new technique to finding organisms and discovered dozens of new phyla of bacteria, practically revolutionizing the tree of life. The Banfield team divided the 789 organisms into 35 phyla, 28 of which were newly discovered, within the domain bacteria. They based the sorting on the organisms’ evolutionary history and on similarities in their 16S rRNA genes; those that had at least 75 percent of their code in common went into the same phylum. The team found vastly different symbiotic species at each level and in each season.
The only way to settle the question was to put people on Mars.
In the fall of 2016 the Banfield team found new bacterial groups from a single Colorado aquifer, doubling the number of the planet’s known bacterial groups, a massive underground find that again revised the tree of life. Banfield also studied the microbial colonization of the guts of infants, taking her into neonatal intensive care units. Her work, and that of others in Yellowstone, in Chile’s Atacama Desert, in Colorado’s and California’s abandoned mines, and even the inside of a dolphin’s mouth, led to a new tree of life published in Nature Microbiology; about a thousand previously unknown species had been added over the past 15 years. A second surprise in Banfield’s discoveries was that almost half of the new bacterial diversity came from one group thought to live only in symbiosis.
Then Nora Noffke ignited the Mars search for life.
Through the hot coastal Virginia summer, Old Dominion University’s Nora Noffke was studying the Curiosity rover photos from Gale Crater. Noffke was a leading authority on microbially induced sedimentary structures (MISS), a term she coined for the stone patterns left by microbial mats in briny shallows. Many people were familiar with stromatolites, the sediment mounds deposited by ancient microbes, but few understood the significance of the microbial tidal mats. Over a 30-year career Noffke had traveled to five continents studying and categorizing more than a dozen typical mat shapes, from roll-ups to ripple marks to wrinkle structures. The moundlike stromatolites made tourist destinations in the shallows off Australia and Hawaii, and in the Caribbean. Noffke found her MISS in the extreme outback of Australia. Virtually no one else had seen them, and they so far ranked as one the oldest pieces of evidence of life on our planet.
In the summer of 2014, NASA invited Noffke to speak at a meeting to choose the landing site for its 2020 rover. If early Earth and Mars were similar, Noffke said to the group, perhaps there were microbially induced sediments preserved on the planet. In the audience, the Caltech geo-chemist Ken Farley sat up in his chair. His team had just published a paper on structures seen by Curiosity in the ancient mud of Mars’s Sheepbed Formation, a coastal plain on the route as it traveled 22 kilometers to Mount Sharp. After the meeting Farley sent Noffke the image and asked her what she thought. She studied the photos taken on Sol 126, the Mars day that Curiosity was in Sheepbed. Her heart leapt. The images looked familiar. She knew the dangerous tendency to see microbial structures everywhere. “I’ll submit a hypothesis paper and see what people say,” she thought.
These strange, previously unknown microbes depended on communities of other organisms to survive.
When, in January 2015, she published a paper suggesting signs of microbial life on Mars, the Curiosity team reacted strongly. Noffke was seeing the equivalent of “clouds in the sky,” said one scientist. The team created a website to refute Noffke’s claims.
The 2014 methane bursts could have been contamination from the Curiosity. But methane is also a signature gas created by microbes like Archaea. Then came the observation in 2015 by the Mars Reconnaissance Orbiter of briny water running in the slopes around Hale Crater in subfreezing temperatures and kept liquid by perchlorate salts.
The rancor grew. The Curiosity team said she had the geology wrong. Noffke responded. “It’s now an eroded hillside but it has been a former lake, in a completely different paleoenvironment. They say it’s a braided river. That’s simply not true. It’s a hillside left from a meandering river system. That’s exactly where you would have microbial mats on Earth, in places like that!”
The only way to settle the question was to put people on Mars. The big problem was carrying enough fuel for liftoff from the Martian surface so that they could return to Earth. For that reason, a first step would likely be humans in orbit around Mars, as proposed by the Planetary Society, presided over by Bill Nye. Looking further, NASA was planning to send humans to Mars as early as the 2030s, while the European and Russian ExoMars mission, scheduled to land in 2020, was to select a lake bed for its site.
Further evidence of ancient microbial activity in the Gale Crater basin came from the University of Oregon, where the geologist Greg Retallack noted the soil’s high level of sulfate, which could only be produced in an anoxic environment by anaerobic bacteria. Some of the “vesicular structures,” or bubbles, in the Curiosity photos resembled those produced by microbes on earth after a rain, Retallack wrote in Geology. For her part, Noffke felt bruised by her experience with big-ticket science and the public fascination with extraterrestrial life. Her paper was merely a hypothesis, not a full-blown argument or claim, and the hostility of the Curiosity response took her aback. The Curiosity team did, however, shape a new itinerary for returning to the site of the methane burst, at exactly the same season as the first was detected. Perhaps it showed, more than anything else, how important the research was becoming to a popular base that included Twitter and Instagram followers like me.
For that reason, researchers and fans eagerly followed the European Space Agency probe Schiaparelli as it winged into orbit around Mars in the fall of 2016 and prepared for its test rover’s descent. Monitored by its orbiting parent unit, the Schiaparelli test lander descended into the Meridiani Planum region on Oct. 19, 2016. The parachute deployed 12 kilometers up, and the heat shield was released at 7.8 kilometers, as planned. Then a mistake happened with its inertial measurement, which ran one second too long as it became saturated with data and generated an estimated altitude that was below ground level. The one-second error triggered the second parachute to fire too soon, as did its braking thrusters, causing the dummy lander to crash hard and disintegrate. The debris could be seen from NASA’s Mars Reconnaissance Orbiter.
The test was a bitter disappointment, but it was only a test. The European Space Agency plans to return to Mars in 2020.
Ted Anton is a professor of English at DePaul University. He is the author of The Longevity Seekers and has written for Chicago magazine, the Chicago Tribune, and Publishers Weekly.
Reprinted with permission from Planet of Microbes by Ted Anton, © 2017 by the University of Chicago Press. All rights reserved.
Original Lead Image: HelenField / Shutterstock