The Indian-American astrophysicist Subrahmanyan Chandrasekhar once remarked that black holes, regions of spacetime whose gravitational field is so strong that not even light can escape its clutches, are the simplest, most perfect macroscopic objects in the universe. Yet that simplicity hasn’t prevented the universe from becoming populated with a veritable zoo of black holes. They appear to range in mass from a few times to many billions of times more massive than the sun.
Stellar mass black holes, the ones formed by collapsing stars, are thought to be the most abundant. Occasionally they get trapped in each other’s gravitational pull and merge in a process of spectacular cosmic violence. It was through such a “binary merger,” about a billion and a half light years away, that humanity first managed to tune in on gravitational waves, the elastic rippling of spacetime that radiates away the energy released by the merger. Black holes thousands of times more massive could have formed in the early universe, when exotic objects called quasi-stars were thought to abound, powered not by nuclear reactions but the infall of hot gas towards a rapidly growing central black hole seed. More massive still are supermassive black holes, with masses up to billions of times that of the sun, thought to be the gravitational anchor at the center of larger galaxies like ours. How these form remains something of a mystery.
Dark matter might be nothing other than a swarm of primordial black holes clustered in haloes around every galaxy.
Interestingly, if one were to attempt a cosmic black-hole census, one would expect the numbers of black holes to thin out drastically in the range of 30 to about 70 solar masses. Our current understanding of stellar evolution puts a cap on the size of the biggest stars, and as a result, the size of black holes that could form in the aftermath of their death (by supernovae).
It comes as something of a surprise then that, since it began observing, the Laser Interferometer Gravitational-Wave Observatory, or LIGO, has detected multiple mergers of black holes precisely within this mass range. Over the last several years, many astrophysicists and cosmologists have been puzzling over where these black holes might have come from. As often happens in research as we fumble our way towards a deeper understanding of the universe, it may come down to some subtlety in our models of stellar and galactic evolution that we’ve yet to contemplate. However, a more striking possibility has recently been revived: That we may need to admit another member to the cosmic zoo of black holes—primordial black holes—the black sheep of black holes, first acknowledged as a theoretical possibility in the late 1960s by Soviet astrophysicists Igor Novikov and Yakov Zeldovich. They realized that these black holes might have abounded long before the stars even had time to form. Might these be the very black holes that the LIGO collaboration has seen?
The possibility follows from the fact that black holes needn’t have formed via some astrophysical process. Black holes can form even in the absence of any matter, from large enough distortions of spacetime that curl up into a singularity. The late physicist Stephen Hawking, knowing this, entertained a breathtaking and simple idea, grounded in the fact that the Big Bang itself was the final stage of an explosive process, one that set up a highly energetic, dense, thermalized initial state that was incredibly smooth and homogeneous—but for the tiniest of microscopic spacetime fluctuations. Hawking suggested that black holes could have formed from these very seed quantum fluctuations that would go on to form, after billions of years, the filament-like scaffolding around which all galaxy clusters now coalesce.
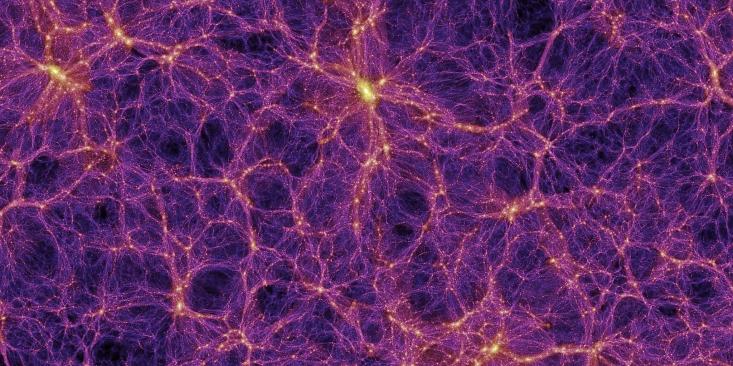
If, somehow, some mechanism dialed up the volume of this primordial noise at small scales (or high frequencies), there would be small but extremely deep troughs in the spacetime continuum that could have, by themselves, collapsed into black holes early in cosmic history, with a wider range of possible masses than those produced later in the universe’s history. This irreducible quantum noise is, of course, the consequence of Heisenberg’s famous uncertainty principle at work in the early universe. According to the principle, one can never know, for example, the position and the velocity of any particle simultaneously. Microscopic particles can therefore never be exactly at rest, as to do so requires simultaneously specifying positions and velocities exactly. The same is true for localized excitations of spacetime itself, which, as a result, fluctuates at all scales in the early universe. This is the noise that seeded the structure of the universe at the largest scales. Hawking realized that any mechanism that amplifies this noise at smaller scales could just as readily produce black holes shortly after the Big Bang.
As appealing as the idea may be, for now primordial black holes remain in the realm of theorists’ imaginations. That could change, of course, if at some point in the near future a gravitational wave observatory sees the merger of a black hole with a mass that could only be primordial. Clinching evidence could take the form, for example, of spotting a black hole with a mass less than about one and a half times that of the sun, the so-called Chandrasekhar limit, named after Subrahmanyan. Such a black hole would be impossible to produce from the collapse of a stellar object, as the lightest possible progenitor to a stellar black hole would not have enough mass to collapse into a black hole below this limit. Although slightly heavier than the Chandrasekhar limit, a recent observation of what would be the lightest black hole (or the heaviest neutron star) ever seen is puzzling enough to have some astrophysicists sanguinely speculating on its primordial origins.
There’s no reason to think primordial black holes can’t be found in our own galaxy, let alone our solar system. In 2015, a pair of Caltech astronomers suggested that a Neptune-sized planet could be orbiting the sun far beyond Pluto, which would explain some peculiar orbits of other more diminutive objects in the Kuiper Belt. But, as a 2019 paper posted on ArXiv suggests, this planet nine could also be a primordial black hole the size of a grapefruit. Some have even gone as far as to posit that dark matter itself—which has eluded every one of our attempts to detect it directly—might be as elusive as it is because it is nothing other than a swarm of primordial black holes clustered in haloes around every galaxy.
Even if dark matter isn’t made up entirely of primordial black holes, their possible existence is a provocative prospect to astrophysicists and cosmologists, not just for their implications for the evolution of the universe, but also its genesis. They could have produced distortions in the relic radiation of the Big Bang through Hawking evaporation. They could have produced the progenitors of the super massive black holes at the center of every galaxy. Their existence could provide a window into the mechanism that set the initial conditions for the Big Bang. For the time being, they remain stubbornly difficult to rule out, keeping many cosmologists and astrophysicists, the author included, preoccupied by their possibility.
Subodh Patil is a theoretical physicist at the Niels Bohr Institute at the University of Copenhagen. He tweets occasionally at @_subodhpatil.
Lead image: NASA