Last summer, in the early days of a heat wave that would culminate in the highest temperatures ever recorded in Paris, I biked across the city to meet my friend Romain Graziani. At a sidewalk café, we sipped burnt espresso and watched the air shimmer weirdly over the cobbles. Romain, who is a scholar of ancient Chinese texts, shared an idea that had emerged from his research: There are certain goals that are most effectively pursued by not striving directly toward them. Like falling asleep, for instance. If you try hard at it, you will not succeed. The same could be said about making a lay-up in basketball, hitting a backhand in tennis, or looking natural in a photograph. There are also, Romain suggested, examples that are more profound and consequential.
Several days later, the mercury had climbed a few degrees higher, and I was on the phone with Phil Taylor, a biogeochemist who has largely abandoned his academic career to work toward reform in industrial agriculture. He was telling me about the great quantity of carbon emissions that can be avoided when broad-acre farmers choose to plant perennials instead of annuals. The roots and microbes of perennials keep organic carbon and nitrogen locked in, whereas the incessant tilling required to grow annuals lets those precious compounds escape.
What if we had a different ur-myth about energy and order in the universe?
“These diligent farmers hit the soil so often and so hard,” Taylor said, “the flywheel of ecology can’t get spinning. They’re just so damn hard-driving.”
After the call, I tried to turn back to my writing. I happened to be working at the time on a book chapter about classical thermodynamics. You might remember something about the augustly named “Laws of Thermodynamics” from a high-school physics course. As usually presented, these laws say physical systems naturally dissipate energy and flow toward a state of cold disorder. From where I sat now, however—in an intolerably hot attic, peering out at a three-dimensional puzzle of zinc roofs gleaming in the sun—it was hard to feel the intuition behind such thermodynamic legislation. To the contrary, for the first time it struck me: What a remarkably strange view of the world. And I found myself wondering, in something of a fever-dream: Where did we ever get such a notion? And might it have something to do with why we’re so damn hard-driving, even when there could be another way?
By returning to the Victorian origins of the laws of thermodynamics, we can see how—and, perhaps, why—those laws have been broadly misconstrued and misapplied. In the 19th century, the first textbooks on the science of thermodynamics emerged from the work of Rudolf Clausius, in Berlin, as well as William Thomson (often called Lord Kelvin) and William Rankine, both in Glasgow. Studying how machines, such as steam engines, could exchange heat for mechanical work and vice-versa, these physicists learned of strict limits on efficiency. The best a machine could possibly do was to give up a small amount of energy as wasted heat. They also observed that if you had something that was hot on one side but cold on the other, the temperature would always even out. Their results were synthesized in the first two laws:
I. The change in the internal energy of an isolated thermodynamic system is equal to the difference between the heat supplied to the system and the amount of work done by the system on its surroundings.
II. Heat cannot spontaneously flow from a colder body to a hotter body. Or, phrased in terms of Clausius’ new concept of entropy, the total entropy of an isolated system will increase over time.
Thermodynamics was extremely useful science for a society in the throes of rapid industrialization and a shift toward a capitalistic free market. The laws and their extensions could be applied to improving the engines driving advances in productivity. Just as importantly, they could be phrased in broad terms that were ideologically aligned with the cultural transformation underway, from an agrarian community of smallholding farmers to an urban society of wage-earning factory workers. For example: You never get anything—energy or work—for free. (On a small farm, in a hospitable climate, you sometimes do; as a factory-worker, you definitely don’t.) Or: Without the input of energy through work (and perhaps combusting coal) a system tends naturally toward cold and disorder. I do not mean to imply that there was something scientifically dubious about the research behind the laws, only that it was neither accidental nor inconsequential that they developed amid—and were articulated in terms reflective of—the broader social transformation that was underway.
Let’s add a fourth law: There is no such thing as an isolated system.
An illuminating parallel for such social embeddedness can be found in Darwin’s work. Many historians have noted the intimate connection between the theory of natural selection and the ascendant political and economic views of the society in which Darwin was a well-placed member. The Victorian elite were committed to the idea that the unfettered competition of Adam Smith and David Ricardo’s free market would drive economic and social advancement. In Darwin’s theory, they found nature’s own reflection of the social system they favored. As J.M. Keynes put it, “The principle of the survival of the fittest could be regarded as a vast generalization of the Ricardian economics.”
Yet while this observation has often been made in relation to Darwin’s work, the theory of thermodynamics has largely escaped similar contextualization. Perhaps physics, in all its rigors, is deemed less susceptible to social involvement. In truth, though, Darwinian and thermodynamic theories served jointly to furnish a propitious worldview—a suitable ur-myth about the universe—for a society committed to laissez-faire competition, entrepreneurialism, and expanding industry. Essentially, under this view, the world slouches naturally toward a deathly cold state of disorder, but it can be salvaged—illuminated and organized—by the competitive scrabble of creatures fighting to survive and get ahead.
As with Darwin’s work, the extension of thermodynamic theory into areas where it did not strictly apply has often led to faulty conclusions. William Thomson himself stumbled into this pitfall when he tried to estimate the age of the Earth. It was a fraught and momentous question—How old is the planet?—because Darwin had just published On the Origin of Species, and the evolutionary process he had proposed required great stretches of time to produce the diverse and exquisite structures of plants and animals. In truth, no one had yet developed those elements of evolutionary theory that permit a well-informed estimate of how long the evolution of life on Earth must have taken. Yet somehow, Darwin had a sense, and he figured it must have taken at least a billion years.
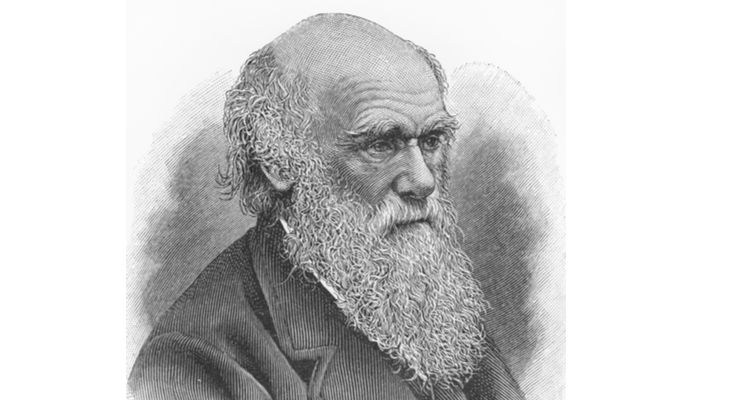
Charles Darwin and Lord Kelvin are invariably memorialized as white-bearded, beetle-browed sages; it is as if they were only ever old. But at the start of this debate about the age of the Earth, Darwin was 50 and Thomson under 40, decades away from being ennobled as Lord Kelvin. Nonetheless, Thomson was already recognized as a founder of thermodynamics, a theory that was quantitative where Darwin’s was narrative, and predictive where Darwin’s was descriptive. A number from Thomson, young as he was, would carry a weight of authority.
Based on measurements made in mines, Thomson knew that the deeper you went, the hotter it got—precisely the observation one would expect if the Earth had once been very hot and had been cooling off ever since. The flux of heat from a hot sphere was a process thermodynamics could describe with precision, so Thomson wrote down a model and asked: If the globe had once been molten, how long would it take to cool to its present temperature? Between 20 and 400 million years, he calculated. The wide range indicated a great deal of uncertainty, but even so, Darwin was aghast. Thomson’s estimate was between two and 50 times smaller than what Darwin figured natural selection needed. Something had to be wrong, Darwin wrote to his friends, but he didn’t know what was wrong—and this was Thomson he was contradicting.
In fact, of the three great sources of heat in the Earth’s interior, Thomson had underestimated one and missed two altogether. The one he had underestimated was the heat originally generated by the planet’s formation—the process of growth by aggregation, which had been driven by energy embedded in the gravitational field. The two sources of heat he had overlooked completely were the friction generated by the flow of molten material inside the Earth and, more famously, radiation. Thomson had no way of knowing that certain elements in the Earth steadily leaked energy, for the phenomenon of radioactive decay had not yet been discovered. To this day we do not know exactly how important this omission was, because we do not know how much radioactive potassium, uranium, and thorium is hidden deep in the Earth. But we are sure it is enough to add significantly to Earth’s core temperature.
Broadly speaking, Thomson overlooked great stores of energy and sources of heat. Consequently, he thought the Earth had cooled a lot faster than it really had, leading him to underestimate the planet’s age. It was the first law, the one that defines a complete accounting of energy in a closed system, that Thomson himself overextended. But the same basic error—energy overlooked—has led to a great many mistaken applications of the second law, as well.
Phrased frequently—and loosely—in terms of disorder and its tendency to increase, the second law of thermodynamics has been invoked to explain why organisms age, why societies collapse, and why evolution requires divine intervention. Leaving aside the problems with thinking of thermodynamic entropy as disorder, the important and basic point is that input of energy can reduce entropy (or disorder), and energy, as we’ve seen, is absolutely all over the place. The energy-driven reduction of entropy is easy to demonstrate in simple laboratory experiments, but more to the point, stars, biological populations, organisms, and societies are all systems in which energy is routinely harnessed to generate orderly structures that have lower entropy than the constituents from which they were built. There is nothing physically inevitable about increasing entropy in any of these systems.
Thermodynamic theories served a society committed to laissez-faire competition.
In almost every erroneous overextension of the first and second laws, one word marks the crux of the error. The laws refer to an isolated system. The idea is that nothing enters or leaves, comes or goes, without being accounted for. We can create models of isolated systems in a laboratory, but even these are only approximations—and not only, as our first thought might be, because a bit of energy is bound to sneak away. They are inevitably approximate also because our world is brimming with energy: There is residual heat that can never be perfectly removed. There is energy sequestered in nuclei by stars and cosmic radiation left over from the Big Bang. There is a ubiquitous energetic fizz of quantum pair production, which may be responsible for driving the universe’s accelerating expansion. And certainly, in everyday life, outside the hermetic enclosures of a laboratory, we are never even close to the realm of isolated systems.
For these reasons, we might consider adding a law. The first and second we’ve just seen, and the third slot is taken by the relation between temperature and entropy, so let’s call this the fourth law of thermodynamics:
IV. There is no such thing as an isolated system.
Granted, our new law places draconian limits on the strict applicability of the first and second, but they will remain as useful as ever for calculations of the idealized or approximate variety. We’re just keeping in mind that they are never exactly right. This is important, because for all the overextensions of thermodynamics that are flagged by explicit reference to the first or second law, there are others in which our underlying sense of the world, shaped as it is by popularized versions of Victorian science, leads us dangerously astray.
Thinking of energy and order as naturally dwindling scarcities that we must drum up through our ingenuity and hustle—or, perhaps it would be more accurate to say, feeling this way about them—it is difficult for us to comprehend and respond to a historical moment in which the gravest threat is not scarcity but superabundance. Not that we will run short of energy, but that we will burn ourselves up in various ways.
Climate change is the obvious example. In our industrious activity, we are utilizing so much molecularly stored energy that we are altering the physical chemistry of the atmosphere. But there are other examples, too. The manufacture of industrial fertilizer can be thought of in energetic terms. Great quantities of energy, mainly in the form of natural gas, are used in a reaction that breaks the extremely stable triple bond of atmospheric nitrogen, N2, in order to produce a molecule where nitrogen resides in a less stable, higher-energy state. The problem is that these newly minted molecules of reactive nitrogen are in enormous excess all over the place. They flow into the ocean, hyperfertilizing cyanobacteria, which bloom, consume all available oxygen, and create large dead zones. From poisoned water at the surface, the reactive nitrogen sinks to the bottom, and when this water cycles back up, decades later, the molecular products of all that nitrogen will be released into the atmosphere. These products—mostly nitrous oxide—are roughly 300 times more effective at trapping heat than carbon dioxide. In short, we are loading the deep ocean with a ticking climate bomb, which our children and grandchildren will somehow have to defuse.
What if we had a different ur-myth about energy and order in the universe? Imagine, for instance, that our intuitions were still informed more by that world of agrarian smallholders, and less by the industrialized cultures that eclipsed it. Letting our worldview shift further still, we could combine such a retrieval of our deeper past with an appreciation of the highly modern. Imagine, that is, a sense of energy informed not by Victorian science but by more recent physics—from cosmic background radiation to the quantum effervescence of space itself. And imagine, too, a feeling for order that derived less from loose notions of entropic decline, and more from recent work on physical self-organization or the way organisms adaptively harness tiny changes in entropy to drive productive chemical reactions.
Imbued with such a worldview, moved and directed by it much as the Victorians were influenced by thermodynamics and Darwinism, we might think and act less in the vein of industrial agriculture, with its mining of natural gas and unidirectional spill of energy. We might be more inclined to let the flywheel of ecology get spinning and investigate the potential allowances of regenerative agriculture. More generally, perhaps we would begin to see that part of the solution to our planetary crisis lies not in hustle, but in repose. Not in catching the next trans-Atlantic flight to an important conference or meeting, but in staying home, contentedly. Not in buying a new more efficient car, but in leaving the old one parked. Perhaps there are many solutions that do not even occur to us, because they resemble passivity rather than action, and we are so deeply trained to hustle.
Aaron Hirsh is a writer and biologist with strong interests in education, evolution, and the environment. Before the heat got much worse, he moved back to Boulder, where he now lives with his family. For the record, he thinks very highly of both Darwinian and thermodynamic theory.
Lead image: Rohane Hamilton / Shutterstock