To a physicist, perfect quiet is the ultimate noise. Silence your cellphone, still your thoughts, and muffle every kind of vibration, and you would still be left with quantum noise. It represents an indeterminacy deep within nature, bursts of static and inexplicable motions that cannot be gotten rid of, or made sense of. It seems devoid of meaning.
Considering how pervasive this noise is, you might presume that physicists would have a good explanation for it. But it remains one of the great unsolved problems in science. Quantum theory is silent not just on where the noise comes from, but on how exactly it enters the world. The theory’s defining equation, the Schrödinger equation, is completely deterministic. There is no noise in it at all. To explain why we observe quantum particles to be noisy, we need some additional principle.
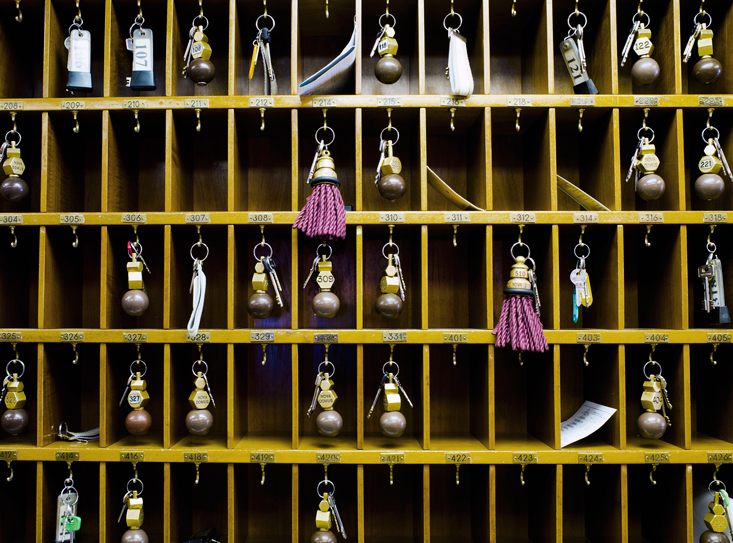
For physicists in the Niels Bohr tradition, the act of observation itself is decisive. The Schrödinger equation defines a menu of possibilities for what a particle could do, but only when measured does the particle actually do anything, choosing at random from the menu. Identical particles will make different choices, causing the outcomes of fundamental processes to vary in an uncontrollable way. On Bohr’s view, quantum noise cannot be explained further. It is what physicist John Wheeler called “an elementary act of creation,” with no antecedents. Genesis was not a singular event in the distant past, but an ongoing process that we bring about. We create the world by observing it.
To skeptics such as Einstein, that view is both wonderfully romantic and completely incoherent. Who are “we”? What is “observing”? Physicists and philosophers have spent the better part of a century seeking a less hand-wavy explanation, taking one of two general directions. Maybe quantum noise, like the noise we encounter in daily life, has a meaning that escapes us. It may seem indeterministic, but could be produced by deterministic processes that, for whatever reason, we can’t see. It might, for example, be a consequence of living in one of countless parallel universes and not being able to tell which is ours. The noise, in essence, tells us where we live. All those little upticks and swerves in particle behavior are the quirks that differentiate our universe from others, and they are “noise” inasmuch as our location is pure happenstance, like being put in hotel room 314 rather than 159.
The noise, in essence, tells us where we live.
The other basic approach is that quantum noise really is meaningless and quantum theory is as indeterministic as Bohr took it to be, in which case the challenge is simply to tidy up the ill-defined concept of observation. In 1986, three physicists—GianCarlo Ghirardi, Alberto Rimini, and Tullio Weber—proposed that not only is quantum noise meaningless, but experimenters don’t trigger it. In fact, nothing does. It shows up, completely unprompted—perhaps once every 100 million years for an individual particle.
Within the interpretational debate, the GRW theory and its variants play a special role. Although they are not the only indeterministic interpretation of quantum mechanics, they are the only interpretation that exposes the indeterminism for all to see—as noise—rather than bury it at a subquantum level. GRW is also one of the few interpretations that is testable empirically. Here, at last, is a data-driven test for a debate that hails from the days of Democritus and Plato: Is the universe at its root deterministic or not?
The GRW theory supposes that noise sporadically strikes particles and causes them to materialize in one of the locations open to them. This can’t happen very often, or else particle behavior would deviate from the Schrödinger equation all the time. Once every 100 million years is enough, because when the blow does come, its effect is greatly amplified by quantum entanglement—the spooky interconnection of particles. A hit on one particle is felt by all those it is entangled with.
This multiplier effect would neatly explain why we observe quantum behavior on the particle level but not in everyday life—why a particle can have no determinate properties but a macroscopic object always does. Although a person or a planet might exist fleetingly in an indeterminate state, just like an isolated particle, it presents a much bigger target and one of its particles will quickly get struck by noise. The noise will fix the location of that particle and all its entangled partners. An object composed of 1023 mutually entangled particles will be pinged every 10 nanoseconds or so.
The noise acts on spatial position, but also affects other properties indirectly. Schrödinger’s famous both-dead-and-alive cat is quickly forced to be either alive or dead, because those two conditions correspond to different spatial arrangements of particles in the cat’s body, and the GRW mechanism will select among them.
The GRW mechanism also demystifies observation. To observe is simply to correlate a particle with some large piece of apparatus, so that the particle’s properties become accessible to you. In so doing you expose the particle to the noise that strikes the apparatus. You may not create reality directly, as Bohr thought, but do make it possible for the all-pervading noise to act on objects it would otherwise pass over.
The general thinking has been that entropy increases because there are more ways to be disordered than ordered.
The GRW theory, like quantum mechanics itself, is a mathematical formalism and does not prescribe what the world is made of—what philosophers call the ontology. Its creators originally envisioned a particle ontology: an atomistic world. But the theory also works if the universe consists instead of smeared-out matter or force fields. What distinguishes GRW from other interpretations of quantum mechanics is that the noise is untriggered. The hits just happen spontaneously. So, they do not strictly require the existence of anything else. The theory thus opens up a wholly new possibility: that the universe is made purely out of noise.
In this radically minimalist view, proposed by the Irish physicist John Bell in 1987 and later developed by Roderich Tumulka at Rutgers University, the universe is like a darkened auditorium lit up haphazardly by camera flashes: a constellation of disconnected moments rather than stuff with an enduring existence. Because those random moments include all our observations, we could never tell the difference between this and a continuous universe. We may see a particle in one place and then, later, in another, and it’s natural to assume that it existed in between, but maybe we shouldn’t. And if the particle doesn’t exist in between, we don’t really have any particles in the way that term is commonly understood.
This point of view is by no means forced on us, but it has an appealing implication: It is the only known way to resolve the tension between quantum entanglement and relativity theory, short of abandoning one of those theories altogether. Even our most advanced quantum theory, quantum field theory—which was created with the express purpose of reconciling these two theories—doesn’t eliminate the conflict that arises during a measurement or equivalent process. As Einstein realized, if quantum physics is indeterministic, what one particle does instantly affects whatever particles it is entangled with. The effect is subtle—it cannot convey a signal—but the word “instantly” is still supposed to be verboten in relativity. The whole reason they call it “relativity” theory is that the passage of time is relative to a given observer, so there is no objective sense in which a process could be instantaneous. One observer might see an effect, another observer at the same position might not, and paradox would ensue. This contradiction evaporates in GRW because the flashes go off on their own. Nothing causes a flash, not even another flash, so their ordering in time doesn’t matter. Whichever flash comes first, the Schrödinger equation will ensure that it is correlated with subsequent flashes. No mechanism operates in space and time to produce this correlation, so relativity simply doesn’t apply.
Even the people who proposed the flash picture don’t much like it. Without a spatiotemporal mechanism, we seem to lose any hope of a deeper understanding of the correlations and have to accept them as brute fact. But the flash picture is worth thinking about, because it is proof that quantum entanglement and relativity theory are not irreconcilably opposed, and it hints that their reconciliation will require a rethink of what we mean by existence in space and time.
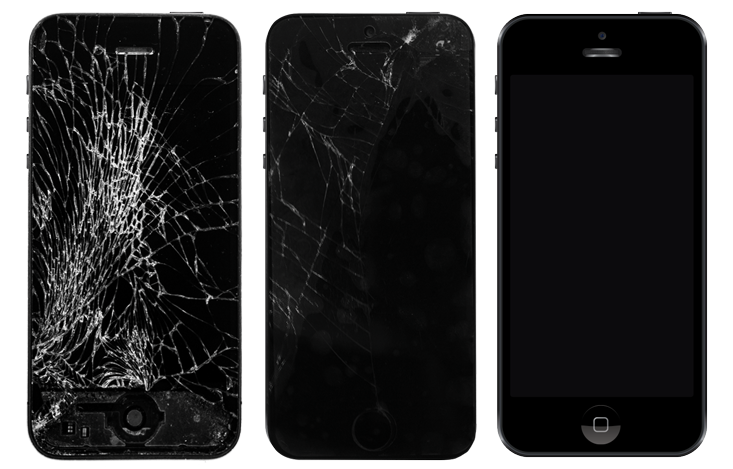
GRW might even solve entirely separate problems within physics. David Albert, a theoretical physicist turned philosopher at Columbia University, thinks it would help explain the second law of thermodynamics: the tendency for entropy—loosely defined as the disorderliness of a throng of molecules—to rise in a closed system. The general thinking has been that entropy increases because there are more ways to be disordered than ordered, so if a system starts off nice and neat, it will naturally degenerate into chaos. But textbooks gloss over an important point: It’s not assured that disorder is more likely. A system can be arranged in an uncountably infinite number of different ways, and there’s no unambiguous way to compare the infinite number of ordered states to the infinite number of disordered ones.
For instance, it’s all too easy to smash your smartphone screen by dropping it on the floor. It is also possible for a cracked smartphone screen to heal itself spontaneously if its molecules move in just the right way. Alas, we never see that. That’s not because such reversals are intrinsically rare. Indeed there are an infinite number of ways for the molecules to reassemble of their own accord. Physicists typically address this problem by fiat: They add a postulate that establishes a convention for counting states, according to which the reversals are rarer.
Instead, Albert suggests, the issue may not be the number of ways, but their distribution through the space of possibilities. Reversals are isolated islands within the sea of states that are on their way to becoming more disordered, and he argues that this geography does not depend on the state-counting convention. Drop a smartphone in slightly different ways and the screen will still shatter. But for a smartphone screen to heal itself, the molecules must approach one another in just the right way, and tiny deviations will spoil that. And this is where quantum noise could play a role. It batters the system incessantly and provides those tiny deviations. If the universe ever goes into reverse, noise will quickly jolt it back to normalcy.
For this to work, the noise must be spontaneous—it must not depend on a trigger such as making a measurement. Moreover, the noise must be truly irreducible—it can’t reflect our ignorance of finer-scale details, or else the entire problem will recur at that finer scale. And the noise should disturb particles by enough to thwart any reversal. Only GRW, among the sundry proposed explanations of quantum noise, satisfies all three criteria.
GRW is a powerful theory, but none of its experimental predictions has held up so far. It should have all sorts of exotic effects. For instance, it could cause an electron to swerve for no apparent reason and emit an X-ray. Catalina Curceanu of the National Institute of Nuclear Physics, in Italy, and her colleagues showed last year that this doesn’t happen more than once every billion years, which rules out GRW’s original estimate. The noise would also buffet gravitational-wave detectors such as LIGO. Because such an effect would depend on the size of the detector, it tests not only how often the noise bursts occur but how large a region they affect. The observed noise in these detectors is extremely low, further crimping the theory.
If the GRW mechanism operates, it has to be more complicated than the original conjecture; for instance, particles’ susceptibility to noise would depend on their mass. If anything, that might be more plausible. So the theory is still alive. That said, the lack of a clear signal is discouraging. If the theory is the standard-bearer for fundamental indeterminism, the null result makes it a bit more likely that the universe, by default, is deterministic at its root.
Determinism wouldn’t strictly eliminate the noise, but merely relocate it. Through the laws of physics, we could trace the origins of each burst of noise—it wouldn’t appear out of nowhere, as in the GRW theory, but would occur because particles had been on certain trajectories. In principle, we could unwind those trajectories back to the initial conditions of the universe, which would consist of all the noise of history, lumped together. Whatever the source of noise, it is the raw material of the world, sculpted into rich patterns through processes of evolution and emergence.
In the lab, physicists seek to strip away the noise of the world and expose its simple core. But at some deeper level, they strip away signal to expose noise, and pivot a foundational question on it. So is noise really signal? “To God all is signal,” the University of Southern California engineering and law professor Bart Kosko once wrote. If anything, though, the opposite is true: To God all is noise; only to man, who constructs his own meaning, is anything a signal at all.
George Musser is a writer on physics and cosmology and the author of Spooky Action at a Distance and The Complete Idiot’s Guide to String Theory. He is a contributing editor at Nautilus, and was previously a senior editor at Scientific American for 14 years. He has won the American Institute of Physics Science Writing Award, among others.
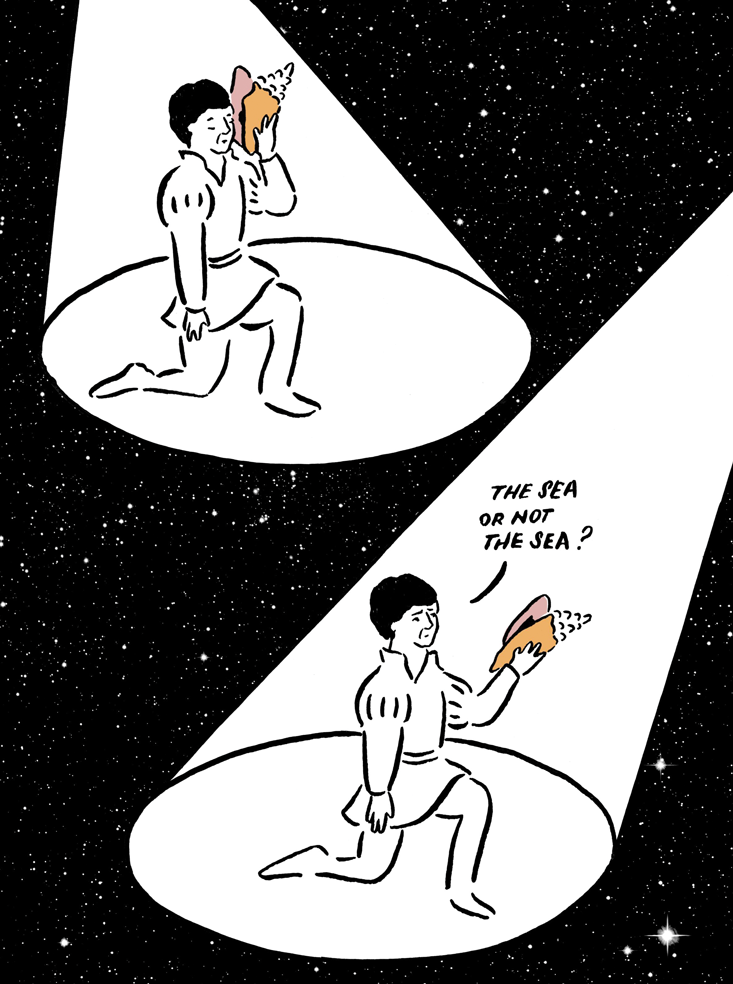