For a theory of the universe as successful as the Big Bang, it may come as a surprise to realize how many complications its promoters had to stumble through. Let’s begin with the unfortunate figure of Alexander Friedmann, the brilliant Russian mathematician and meteorologist who was the first to exploit something remarkable about Einstein’s “field equations,” the set of ten equations that reimagined gravity as an outcome of curved spacetime.
In 1917, Einstein, perhaps to not seem too out-there, argued that one could use the field equations to derive a model of the universe very much like the traditional Newtonian view—an eternally static, or “steady-state,” cosmos. The difference in Einstein’s model was that it was finite in size where Newton’s was infinite.
Universes like Friedmann’s could only be “curiosities,” Einstein said, never true descriptions of reality.
This was too conservative of Einstein, Friedmann thought. He instead viewed Einstein’s model as just one example of many possible universes. So, in 1922, publishing in the leading European science journal Zeitschrift für Physik, Friedmann derived three kinds of cosmological models that were much more dynamic than Einstein’s: One was a finite universe of positive curvature—like a sphere—that expanded from a singularity and then eventually stopped expanding and collapsed back to a point; the second: an open infinite universe of negative curvature, which could be pictured like a saddle of infinite size; and finally: a universe of zero curvature—a flat plane—that expanded at an ever slower and slower rate, coasting into infinity.
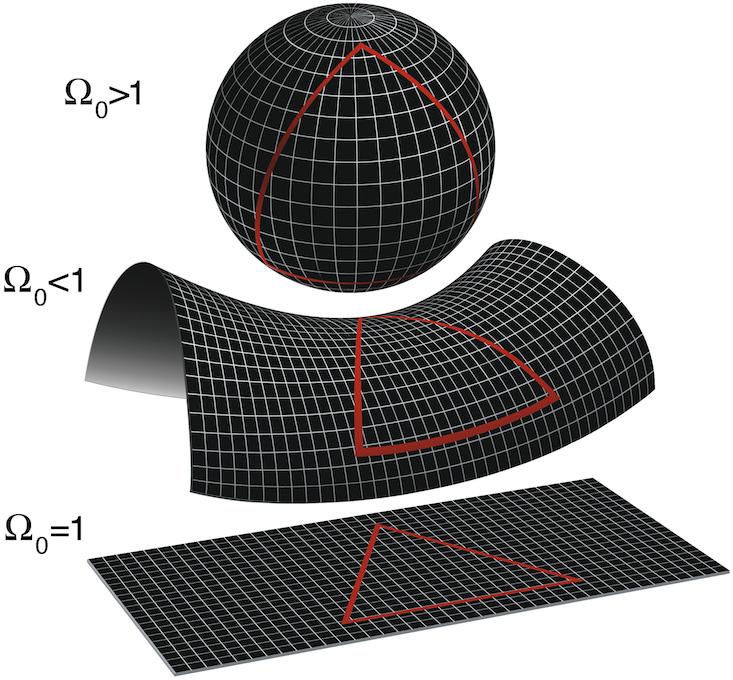
Friedmann’s 1922 paper fell dead-born from the press. But Einstein, once he got around to reading it a year later, was so disturbed that he hastily wrote a rebuttal in which he claimed Friedmann had made a mathematical error. But as Friedmann made clear in his own reply, it was Einstein who had made the miscalculation. Einstein retracted his claim, yet nevertheless maintained his opinion that universes like Friedmann’s could only be mathematical constructs or “curiosities,” as he said, never true descriptions of reality.
For all his ingenuity, that was where Friedmann’s part in the tale of the Big Bang ended. In 1925, after he became director of the Main Geophysical Observatory in Leningrad, the man—just 37 years old—died of typhoid fever not long after performing a record-setting balloon flight. But his ideas would prove indispensable. Not only did he introduce the phrase “expanding universe,” he deserves, some argue, to be known as “the father of Big Bang,” because he was the first to correctly apply general relativity to cosmology, the development of the universe.
Without knowing it, in that same year Belgian priest and physicist Georges Lemaître began following Friedmann’s footsteps—and would later see that Friedmann had it right. But Lemaître was not modeling the Big Bang. Not yet. What Lemaître did was basically to build a bridge between Einstein’s eternally static model and one that could evolve. He posited a static Einsteinian universe at the origin that would then expand and flatten over time.
Lemaître collected a modest number of astronomical observations of distant nebulae before scientists realized they were independent galaxies in their own right. Using these observations, Lemaître very roughly plotted an expansion trajectory showing that the further away a given extra-galactic nebula was, the faster it was receding into distance space. He wrote a paper suggesting this could be taken as evidence of the universe’s expansion.
This was radical. But then for reasons that remain unknown, he virtually guaranteed no one would notice it by publishing in an obscure Belgian journal in French. Too add insult to injury, two years later, in 1929, American astronomer Edwin Hubble made headlines demonstrating an even greater number of galaxies showing recession—their distance from us was proportional to how fast they’re flying away from us. Although Hubble himself was quite conservative about the notion of an expanding universe, he knew cosmologists who supported the idea would welcome his findings.
By now, even Einstein could not shrug them off. In early 1930, he and other physicists met in England at the Royal Society, run by Sir Arthur Eddington, and they tried to agree on a cosmological model consistent with Hubble’s new measurements. When he heard of the meeting a few months later, Lemaître resent his 1927 paper to Eddington, who had been his mentor in Cambridge. Chagrined that he had forgotten all about it, Eddington hastily had the essay translated into English. Here, at last, was an expansion model all could agree on, Einstein in particular: Lemaître’s paper incorporated Einstein’s model as an initial condition of the universe.
But Lemaître wasn’t satisfied. By 1931, he had come to believe that Einstein’s “initial condition” state could not be stable. Reaching back to Friedmann, he proposed his Primeval Atom hypothesis, essentially the Big Bang 1.0, that the universe must have initially started from a fantastically dense kernel and expanded outward. “The Cosmic Egg exploding at the moment of creation,” was how the priest phrased it.
This was too much for Einstein, Eddington, and their other colleagues. They disliked the metaphysical implications of a universe with a temporal origin—it was too tempting for some to see God lurking behind it.
Progress stalled at this point as Nazism dramatically changed the priorities of scientists around the world. And although Lemaître didn’t know it, his own part in the saga, like Friedmann’s, was over. For physicists, work on general relativity and cosmology took a back seat to the war effort and the growing interest in quantum mechanics. Lemaître, confined to Belgium under German occupation (and narrowly surviving Allied bombing), turned his attention to computers and problems in celestial mechanics.
But during this period, the Big Bang idea caught on with George Gamow, the colorful Ukrainian physicist who fled the Soviet Union in the 1930s and came to America. Since he could not work on the Manhattan Project—no security clearance—Gamow busied himself with another puzzle: how the Big Bang might explain the evolution of all the universe’s heavier atomic elements, like iron and gold.
Gamow first studied relativity at the feet of Friedmann in the early 1920s. Once in the US, he returned to the topic and adopted his own pupils—the European refugees Ralph A. Alpher and Robert Herman. He put the two precocious physicists to work on a model of the universe similar to Lemaître’s—except rather than starting with the cold primeval atom, they postulated a hot, super dense state.
Only this, Gamow, Alpher, and Herman reasoned, could allow nucleosynthesis to “cook” hydrogen and helium into iron. One immediate consequence of such a hot big bang model, they pointed out, is that radiation from the primeval fireball—now known as cosmic microwave background radiation—should still permeate the universe, albeit at very attenuated wavelengths. Alpher calculated a temperature to this background radiation of about five degrees Kelvin.
But again, as with Friedmann and Lemaître before them, Gamow, Alpher, and Herman’s paper, published in 1948, was ignored. Perhaps their failure to enjoin their peers to look for the cosmic background radiation had something to do with it. If things had turned out differently, Gamow may not have left cosmology to study genetics in the 1950s, after being inspired by the discovery of DNA. Maybe Alpher and Herman wouldn’t have left academia to move into industry—General Electric and General Motors, respectively.
History shows how the route to scientific recognition was littered with others’ bad luck.
It wasn’t until 1965 that theorists and experimenters finally hit pay dirt. Robert H. Dicke and P.J.E. Peebles at Princeton University, unaware of Gamow’s work with his pupils, figured out the details of a background radiation based on expansion from a fireball state of the early universe. Like Alpher and Herman, Dicke and Peebles predicted a background noise would measure between three and five degrees Kelvin.
They built a radio detector to measure it themselves but, as luck would have it, found that someone else had come across the background noise first. Two physicists working for Bell Labs, Arno Penzias and Robert Wilson, had been preparing a radiometer in New Jersey and stumbled on the signal before they did. Penzias and Wilson thought the “noise” they were detecting was just some earth-based interference muddying their real target: radio signals from the deep space between distant stars.
When they reported on this “noise”—which measured just above three degrees Kelvin—Dicke and Peeples immediately recognized its significance and got in touch with them. The two groups published their research separately. The discovery of what turned out to be the cosmic microwave background radiation was seen to be the strongest evidence for the Big Bang, an echo, in terms of radiation, of the explosion of the early universe.
The landmark finding would win one of these duos the Nobel Prize—can you guess which one? In 1978, the award in physics for that year went to…Penzias and Wilson, not Dicke and Peeples, nor any of the earlier theorists whose hard work laid the foundations for the discovery. Dicke and Peeples felt left out. But Alpher and Hermann were particularly bitter; they felt leaving academia for industry had been counted unfairly against them. Lemaître and Gamow were both long dead.
We can only speculate how different the theory’s progress might have been, had, for example, Alexander Friedmann not died in 1925 before reaching the age of 40. Would he and his then pupil George Gamow have joined forces with Lemaître in the 1930s and developed a hot big bang model much sooner? Would they have predicted the cosmic microwave background radiation years before?
What does appear certain is that, even when the grandest theories of science are beset with unpredictable obstacles, sooner or later someone new comes along to carry forward the torch. It’s just too bad that, so often, it’s those who carry it across the finish line that seem to get all the credit. But history shows how the route to Penzias and Wilson’s recognition was littered with others’ bad luck.
John Farrell is the author of The Day Without Yesterday: Lemaiître, Einstein and the Birth of Modern Cosmology.
WATCH: The Princeton physicist Paul J. Steinhardt on what the Higgs Boson has to do with cosmology.
The lead image is courtesy of CERN via Ars Electronica/Flickr.