Elements that are critical to life—carbon, hydrogen, nitrogen, and oxygen—are also the principle components of the fuels and fertilizers that our civilization depends on. These elements are all found in abundant quantities in the Earth’s atmosphere. In principle, we could make all the fuels we need from air. Indeed, that’s exactly how nitrogen-based fertilizer is made, thanks to the invention of catalysts. A century ago, scientists Fritz Haber and Carl Bosch devised catalysts that enabled a chemical process to “fix” atmospheric nitrogen into a form usable by plants—and ultimately by people via the food we eat. The invention transformed agriculture, which in turn enabled expansion of the Earth’s human population from 2 to 7 billion. If we could apply the same “fix” for fuels, then the result of burning them would simply return carbon taken from the air back to the atmosphere—a sustainable cycle with negligible impact on climate.
The challenge of creating such a fuel “fix” comes from the fact that the small molecules of these elements found in the atmosphere are extremely stable. In water (H2O), the atoms are connected by strong chemical bonds, needing temperatures above 2000 dioxide (CO2) apart. So it’s not surprising that synthesis of nitrogen fertilizers with the Haber-Bosch chemical process requires high temperatures, and consumes 2 percent of all global energy use, mostly from natural gas.
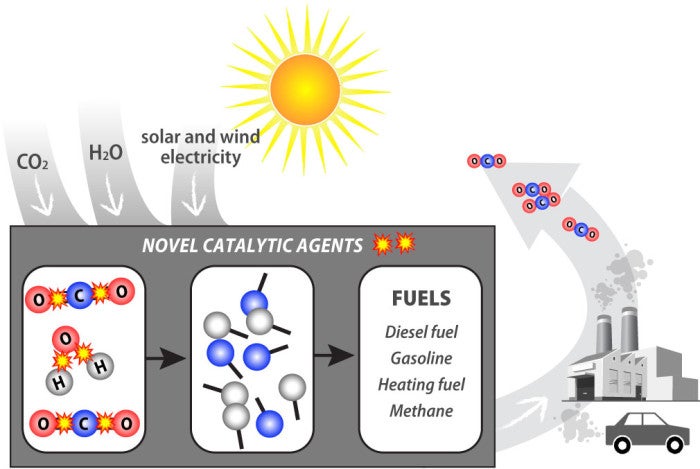
Long ago, nature found ways to solve this problem. In plants and algae, photosynthesis uses sophisticated biochemical catalysts (enzymes) to split CO2 and H2O and use the freed elements to create larger complex molecules that form the ingredients of food (proteins, carbohydrates, fats, etc.) and fuels (hydrocarbons). That’s where the fossil fuels we burn today ultimately come from. But the present scale of our energy and fertilizer use is such that we are putting far more CO2 back into the atmosphere—35 billion tons a year—than is being removed from it. As a result, current and future generations face serious environmental consequences.
The challenge we face is to make ordinary materials do things they don’t normally do.
But what if we could harness scientific creativity to replicate what Haber and Bosch did—only better? Could we split water to liberate hydrogen, carbon dioxide to liberate carbon, and “fix” nitrogen—all without using any fossil carbon-based forms of energy? Can we chemically transform CO2—affordably and at scale—into the source of the carbon that we use as fuels, chemicals, concrete, fiber composites, and so much else? If so, then sustainable carbon and nitrogen cycles for the Earth would be in reach. Meeting this challenge requires three things:
- A source of inexpensive non-carbon energy that can help split the molecular bonds (in fact, electricity from wind energy is almost cheap enough and solar photovoltaic electricity costs are nearly so and trending lower).
- An inexpensive source of carbon-free hydrogen to combine with the liberated carbon or nitrogen (this can potentially come from splitting water, but existing catalysts to activate the chemical transformation are simply not good enough).
- New catalysts that can activate CO2 or N2 and the transfer of hydrogen to form C-H and N-H bonds far more efficiently than any currently available.
The catalysts are the core of the challenge, and trial and error experimentation has not yielded many promising candidates to date. Most conventional catalysts don’t have the ability to distinguish among different intermediate chemical species, or to suppress undesired products during the course of the chemical reaction. The most promising way forward is to mimic nature’s catalysts—enzymes—whose three-dimensional nature makes them more selective and able to overcome these problems. Furthermore, the growing ability to control the composition of materials at nanometer scale—essentially atom by atom—makes it possible to fabricate such advanced catalysts. It’s also now becoming feasible to predict the properties of potential new catalysts from theory, using large-scale computations on the basic equations of quantum mechanics—which should enable scientists to focus on the most likely catalyst designs. So combining theory, nano-scale synthesis techniques, and advanced characterization and testing makes possible a new paradigm for materials design, one that is potentially able to address the challenge of novel and extremely powerful catalysts.
This is an extremely ambitious scientific challenge—to invent artificial cycles for carbon and nitrogen that are efficient and affordable enough to operate on an unprecedented industrial scale. Essentially we are attempting to restore the balance in the Earth’s carbon and nitrogen cycles that has been lost through the exponential increase in the demand for food and fossil fuels—perhaps the most important challenge of the 21st century. We are in the best position ever to undertake this effort, scientifically and technologically. But to do so requires major resources, an unprecedented approach, and urgency: The time to act is now.
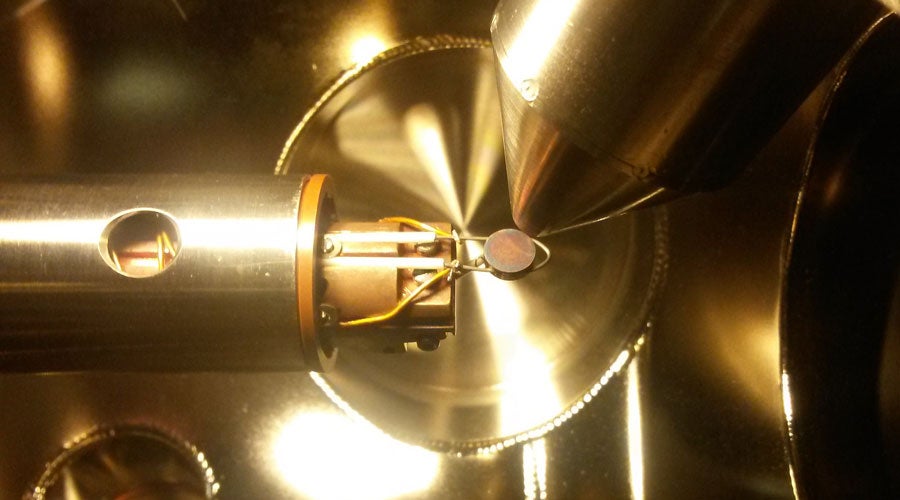
How to Create Novel Catalysts of Unprecedented Power
The research needed to address this challenge involves creation and integration of knowledge from multiple disciplines, including materials science, electrochemistry, photochemistry, computational science, and chemical engineering, among others. The fundamental research should be sufficiently open and unconstrained to explore new materials and energy-matter interactions. But scientific solutions are not enough. We need catalysts that can become part of large scale, affordable industrial technologies—ultimately capable of processing more than 10 billion tons of carbon per year. So the overall effort needs to be guided by a strong sense of engineering practicality. Moreover, since we can’t predict the outcomes of basic research in advance and the challenge is urgent, it makes sense to focus research on several parallel catalytic paths at the same time—thermochemical, electrochemical, biochemical, and photochemical—by investing in a portfolio of approaches. It may even be possible to combine two or more of these catalytic pathways.
Under these constraints, the pace and efficacy of research will likely be highest if teams of three to five researchers collaborate and combine their expertise with very little administrative overhead. Thus we suggest investing in multiple investigative scientific teams, not just in individual investigators. Think of these as the scientific equivalent of a Silicon Valley startup, capable of failing fast, adapting quickly, and innovating rapidly. Moreover, funding multiple teams for each catalytic pathway—also a portfolio strategy—is analogous to the way venture capitalists deploy money, and is likely to increase the chances of success.
The challenge we face is to make ordinary materials do things they don’t normally do. So the investigative teams will need access to unprecedented computational power to do quantum calculations. They will need the ability to synthesize materials with unprecedented nanoscale control—which means ultraclean rooms, high vacuum systems, new kinds of microscopes. They will need to probe the properties of prototype catalysts with ultrafast light beams, x-rays, and other advanced spectroscopy techniques. They will need to test materials and to characterize reaction products precisely with advanced mass spectrometers and other tools. And they will need to design and build first-of-a-kind experimental reactors to show that the catalysts function as expected, and to demonstrate their potential to produce—cost-effectively—large volumes of C-H and N-H chemicals—the building blocks for fuels and fertilizer.
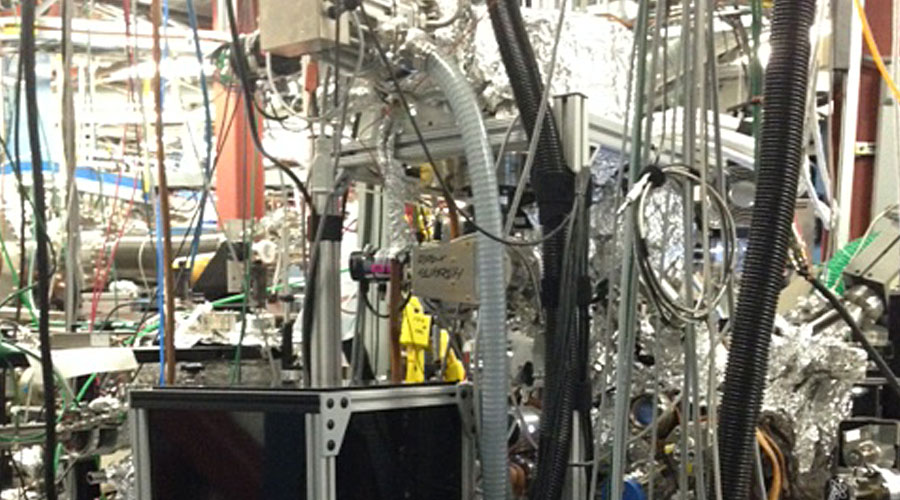
We believe that this effort will cost about $100 million a year for period of 10 years. This level of funding would support between 25 to 50 teams across research universities and laboratories in the United States and elsewhere. Such a level of investment is unlikely to come from federal sources, where science budgets appear likely to remain flat for the foreseeable future and where the portfolio approach proposed here would likely be difficult to realize or sustain. Thus there is an extraordinary opportunity for private philanthropy—for an objective that could be, quite literally, life-sustaining for the Earth.
While the focus of the proposed research is to invent energy-efficient artificial cycles for carbon and nitrogen, it is worth noting that these efforts will likely have broader impacts. Catalysts are widely used in the production of chemicals, pharmaceuticals, plastics, and many other materials. The ability to discover and synthesize novel catalytic materials with properties that are controlled at nanoscale will be useful in many aspects of the economy, and will likely enable many novel and useful products. In effect, this research would lay the foundations for an industrial renaissance that is cleaner, more efficient, and enormously valuable.