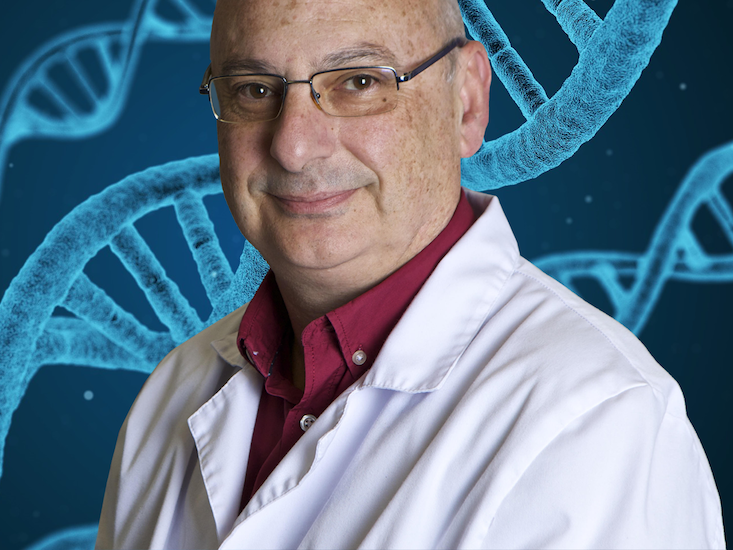
When Francisco Mojica was 25, he supported himself by tracking bacteria in the Mediterranean off the coast of a tourist haven in southeastern Spain. At the time, he was a doctoral candidate at the University of Alicante, where he focused on a much stranger microorganism than those he was searching for in the ocean: Haloferax mediterranei, a single-celled creature that thrives in water so salty it kills almost everything else. “Even sea water is not salty enough for them,” he says.
To understand this peculiar creature, Mojica, his advisor, and another graduate student were painstakingly sequencing bits of H. mediterranei DNA. This was the early 1990s—pre-Human Genome Project, pre-modern genomics—and it was frustrating work. When Mojica found bizarre, stuttering repeats of DNA bases, he assumed they’d screwed up somehow. “It’s impossible that you get exactly the same sequence many times!” he recalls thinking.
But every time Mojica and his colleagues repeated the experiment, the same pattern—30 or so bases that appeared over and over again, separated by lengths of seemingly unrelated DNA—reappeared. Reading journal articles in the library, Mojica learned that a Japanese group had noticed something similar in the genome of E. coli a few years before. Despite the fact that the repetitions did not seem to be connected to H. mediterranei’s predilection for salt, he put a chapter on them at the end of his Ph.D. thesis. And by the time he turned the thesis in, he couldn’t stop thinking about them.
The answer seemed to be stranger and more profound than he had ever dreamed of.
There weren’t many people who shared his interest. Unexplained oddities are common in the genomes of most organisms, from humans to archaea, the group of microorganisms to which H. mediterranei belongs. Even after moving on to other subjects, Mojica remained fascinated by the fact that E. coli and H. mediterranei, which were only distantly related, both had repeats—and that the “spacer DNA” between the repeats was always about the same length despite having a wide variety of different sequences. What were these things for?
In 1994, between a pair of short-term positions, he returned to these single-celled curiosities and inserted extra copies of the repeats and spacers into H. mediterranei to see what would happen. The cells promptly died—“It was amazing!” he recalls fondly—and he wrote a paper suggesting the extra copies interfered with the cells’ ability to reproduce correctly. (He was wrong.) After he was hired to teach at University of Alicante in 1997, he tried, fruitlessly, to see if the same thing would happen in E. coli. Perhaps the repeats formed small loops in the genome for proteins to attach to? (Wrong again.) “Nothing worked,” he says.
Still, in the years since he’d started his work, genome sequencing had gotten much easier. By the early 2000s, other people were starting to wonder about both the patterns that had intrigued Mojica and the genes around them, including Roger Garrett at the University of Copenhagen, Ruud Jansen at Utrecht University in the Netherlands, and Eugene Koonin at the Unites States’ National Center of Biotechnology Information (NCBI). When Mojica and Jansen struck up a correspondence, they began tossing around catchy names for the patterns, and on Nov. 21, 2001, they settled on CRISPR—an acronym for Clustered Regularly Interspaced Short Palindromic Repeats.
Mojica finally found the key that was to unlock the origin of the spacers, and, along with it, the meaning of the puzzle that had transfixed him for so long, in 2003. While studying E. coli, he realized that the spacers were pieces of DNA from viruses that were retained in the genome of the host species—and that some of the microbial strains that carried the spacers were either already known to be resistant to infection or had no record of ever being infected.
One of the genes made a protein that could snip through almost any sequence of DNA.
What if, he wondered, the repeats, spacers, and associated genes didn’t have to do with reproduction or any of the other hypotheses that had been advanced over the years? What if, instead, the repeats were a type of adaptive immune system, used by hosts to recognize attackers by their DNA? Nothing like that had ever been seen, or even looked for, in a microorganism. Mojica was overcome, and found himself with tears in his eyes. It had been 10 years since he wrote about these curious repeats in his thesis. If his theory was correct, the answer seemed to be stranger and more profound than he had ever dreamed of.
In 2005, Mojica and his group published a paper in the Journal of Molecular Evolution with this hypothesis, and the following year, Koonin and his colleagues at the NCBI laid out in more detail just how such a system would work. In 2007, researchers at the Danish-based food and enzyme company Danisco confirmed that CRISPR did indeed represent a microbial immune system. In the following years, groups of researchers around the world described how bacteria and archaea collected viral DNA and how the subsequent destruction of invading viruses worked.
Soon, another crucial breakthrough occurred. One of the most interesting insights related to CRISPR was that Cas9, one of the genes associated with the repeats in bacteria, made a protein that could snip through almost any sequence of DNA. In 2012, the University of Umea’s Emmanuelle Charpentier and the University of California, Berkeley’s Jennifer Doudna led a team that demonstrated how Cas9 could be used to cut and paste genes into the DNA of living cells. Suddenly, a barely imagined age of gene editing was born.
Genetic engineering with CRISPR-Cas9 is one of the most exciting and controversial technologies of the 21st century. Scarcely a day goes by without new stories about its potential utility. It’s behind a novel method for destroying populations of disease-spreading mosquitoes, and researchers are looking to see whether human embryos can be given functioning copies of mutated genes in order to cure crippling or deadly genetic diseases before birth. There’s even speculation that some of the early groundbreaking work to develop the technique, including that done by Charpentier and Doudna, will be rewarded with a Nobel Prize.
For his part, Mojica, whose nagging curiosity helped get things started, still draws a salary as a teacher at the University of Alicante. It’s only when he manages to get some grant money that he can continue to do the research that he loves. “If I don’t get the grant, nothing happens,” he says. “I can do bioinformatics”—working with data that already exists—“or I can go home and grow tomatoes.”
When it’s an open question exactly what your research will be useful for 10, 20 years down the road, it’s difficult to convince funders to take an interest. Mojica understands the attraction of trying to predict practical applications. But his experience has taught him how beside the point that can be. “Basic science is like a tree that gives you apples, pears, pineapples—many different fruits just from the same tree,” he says. “This is an example of that. You do some experiments, basic research, and eventually, you realize the function of these repeats.” This realization—the discovery that microbes have an immune system—is on its own a phenomenal achievement, in terms of understanding biology. “And, afterward, many different applications derive from this discovery.”
He is proud that his strange little repeats turned out to be something after all, and that they provided the kernel for such a transformative technology. He doesn’t use the gene editing technique himself. But he is impressed and thrilled by the work of people who do. “They are doing,” he says, “wonderful things.”
Veronique Greenwood is a science writer and essayist. Her work has appeared in The New York Times Magazine, Smithsonian, Discover, Aeon, and many more.
WATCH: Siddhartha Mukherjee says genetics is challenging our power to interpret our own narratives.
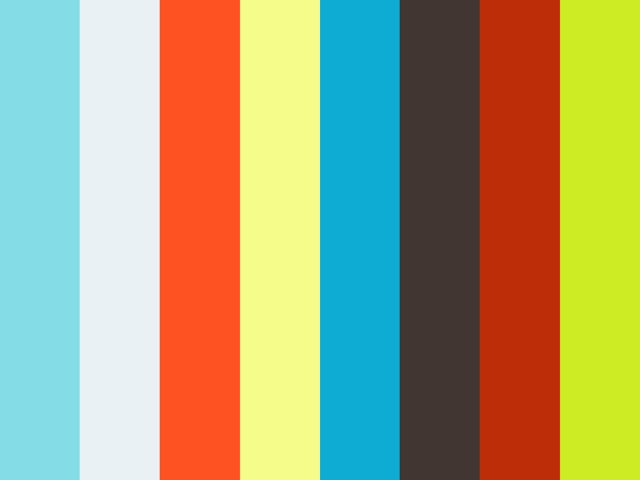
This article was originally published on Science Philanthropy Alliance in March 2017.