Conservationists and bioethicists often regard the packages of engineered DNA called “gene drives” with a mixture of wonder, excitement, and dread. Gene drives violate the normal rules of inheritance by making sure they get passed down to all of their host organism’s offspring, not just to half of them; they therefore have the unnerving potential to rapidly and irrevocably alter a population. Much of the controversy about gene drives has centered on the practicality (and hubris) of using them to control dangerous insect pests, since insects were about the only animals in which gene drives had been shown to work.
That has now changed. In a paper appearing this week in Nature, biologists at the University of California, San Diego demonstrate for the first time that current gene drive technology also works—at least up to a point—in a mammal: the mouse. Their findings highlight the potential, but also the significant limitations, of putting gene drives to work in the real world. For at least some time to come, these kinds of “active genetics” technologies may be more useful as laboratory tools than as instruments for remaking nature.
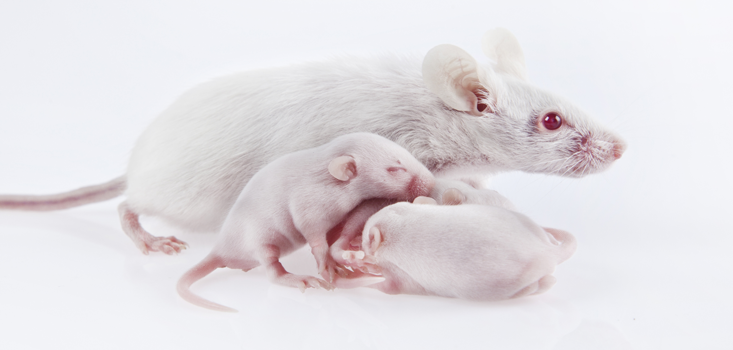
Fred Gould, an entomologist and evolutionary biologist at North Carolina State University, likens gene drives to the fictional substance ice-nine in Kurt Vonnegut’s novel Cat’s Cradle: a bizarre form of ice that freezes all other water it touches. Gene drives spread fast because they are sets of genetic elements that spontaneously copy themselves from a maternal chromosome to a matching paternal one or vice versa. In the process of copying itself, the gene drive can also add, delete, or modify genes at its insertion point. Back in 2003, gene drives were suggested as a theoretical possibility, but the advent of CRISPR/Cas9 genome editing in 2012 suddenly made them much more feasible.
Although a combination of legal restrictions, ethical concerns and fears of unintended consequences has held back gene drives from being tested in the wild, the allure of the hypothetical good they might do has compelled scientists to continue studying them. A well-regulated gene drive could arguably save millions of lives, for example, by rendering Anopheles mosquitoes resistant to infection by malaria parasites.
Interest has also been high in developing gene drives to rein in rats and mice, which as invasive species often acutely threaten wildlife. On Midway Island, a crucial albatross breeding ground in the North Pacific, the birds were nearly wiped out by predatory rats that arrived about a century ago. A massive poisoning campaign eliminated the rats and saved the albatrosses in 1995—but conservationists would rather have a less blunt weapon for stopping unwanted mammals in other situations. (According to reports from a year ago, mice on Midway Island are now becoming a new threat to the birds.)
You could make genetically complicated animals that maybe couldn’t be made before.
Yet what first drew the developmental biologist Kimberly Cooper to dabble in gene drive technology for mice was not conservation but evolution. In her laboratory at UCSD, she and her colleagues study the evolution of the jerboa, a long-legged rodent with a jumping, bipedal gait, which diverged from the mouse lineage tens of millions of years ago. Cooper and her colleagues are trying to find the unknown combination of genetic changes responsible for that evolutionary transformation by making lab mice carry incrementally more jerboa traits.
That kind of project is onerous with mammals, however, because of all that’s involved in getting desired genes into individual animals through conventional breeding. Genetic experiments commonly need animals that are homozygous for a trait, meaning that they carry copies of the relevant gene on both their maternal and paternal chromosomes. To create a mouse that is homozygous for just three mutations of interest, researchers might need to cross hundreds or even thousands of mice with single-chromosome mutations. Cooper’s jerboa project could easily turn out to need mice homozygous for 10 or more genes.
“I always thought that it would be impossible because the genetics of combining multiple things is a mess in mice,” she said. “You need so many animals. It takes so much time and so much money.”
But three years ago she was inspired to follow the lead of her UCSD colleagues Valentino Gantz and Ethan Bier, biologists who had recently demonstrated what they called “active genetics” in fruit flies. Active genetics refers to the use of genetic elements to boost the inheritance of specified traits beyond the normal 50-percent rate that Gregor Mendel first observed; Gantz and Bier classify gene drives as a self-perpetuating form of active genetics.
“I recognized the same technology could be used in the lab to ideally increase the rate of inheritance of an engineered version of a gene,” Cooper said. It would also allow her to bring in genes from species other than mice. “You could make genetically complicated animals that maybe couldn’t be made before.”
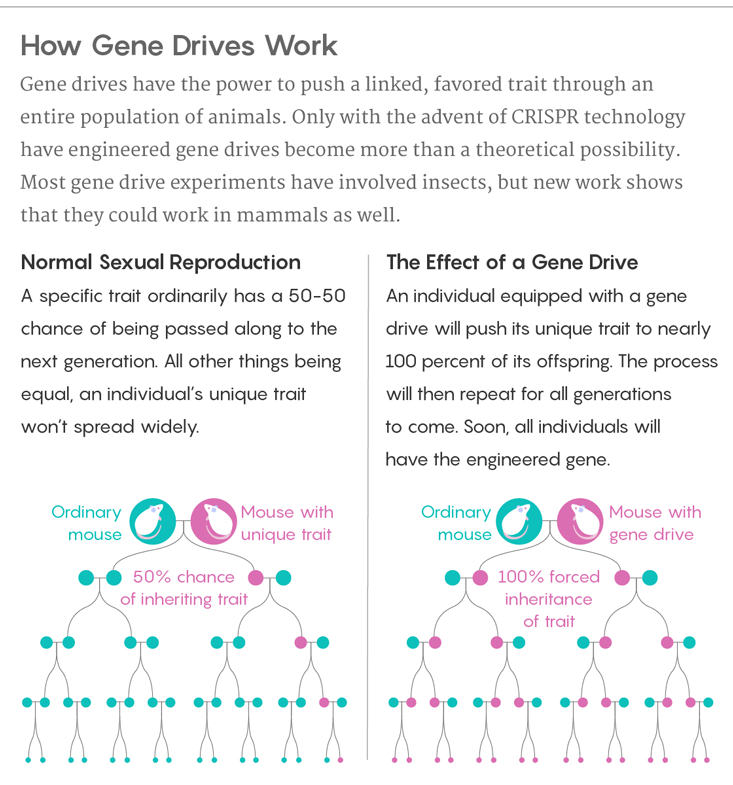
As they describe in their new paper, Cooper and her team engineered an active-genetics mechanism that enabled a “marker” gene to spread quickly through a lineage of mice. Cooper emphasizes that what they made was not technically a gene drive: As a safety measure, the two components of her mechanism—one responsible for cutting the DNA, the other for targeting the cut—were separated into different parts of the genome. This separation prevented the mechanism from spreading through multiple generations uncontrollably. Nevertheless, its success served as a proof of concept for gene drives in mammals.
Even so, that success came with caveats. Unexpectedly, Cooper’s active-genetics system didn’t work in male mice, only in females. And even among the females, the mechanism worked only about 70 percent of the time at best.
“If someone were to try to build a gene drive using this type of mechanism right now, it wouldn’t spread through a population very quickly and probably wouldn’t persist,” Cooper said. The limitation to females would reduce the efficiency of gene drives for developing new lines of lab animals, too, but it could at least speed up the breeding process.
She and her colleagues suspect that the gene-copying mechanism performed differently in males and females because of subtle differences in the ways that sperm and eggs are made. In an egg-making cell, pairs of chromosomes may line up with each other earlier in the production process, improving the odds that if one of the chromosomes gets cut, the cell will repair it with the desired DNA from its partner. “We might be able to improve the efficiency and make it work in males if we can get the timing right,” Cooper said.
Anthony James, a molecular geneticist at the University of California, Irvine, said that, with some improvements, active genetics should eventually be useful for producing the complex lab animals that diverse fields need for their genetic studies, providing a far more efficient alternative to today’s techniques. (James collaborated with Gantz and Bier on an experimental anti-malaria gene drive for mosquitoes in 2015.) Conventional breeding of mice with specific combinations of many genes might have taken a decade in the past, he said; active genetics might shorten that to one or two years.
Inserting genes into mice one by one with CRISPR would be another way to do it, but he believes active genetics will be superior to that, too. “Regular old CRISPR is good for modifying a gene that’s already in there,” he said. “But if you want to add genes, or swap them out and replace them, the gene drive system would be much better for that.”
The enthusiasm expressed by Bruce Conklin, a geneticist at the Gladstone Institutes in San Francisco who wrote a commentary on Cooper’s study for Nature, is more measured. “It’s valuable, but with CRISPR, we can [already] make really complicated models really quickly,” he said. Still, he hails the work for highlighting overlooked differences in male and female cell biology, and he thinks the active genetics approach it champions might aid understanding of DNA repair and other fundamental mechanisms in molecular biology.
Cooper sees a bright side in the fact that gene drives seem to be harder to create in mammals than in insects, given the controversy around their potential use. Improving the efficiency of active genetics in mammals will take some years. “In the meantime, we can have serious conversations about what can and should be done [with gene drives] in the wild,” she said.
John Rennie joined Quanta Magazine as deputy editor in 2017. Previously, he spent 20 years at Scientific American, where he served as editor in chief between 1994 and 2009. He created and hosted Hacking the Planet, an original 2013 TV series for The Weather Channel, and has appeared frequently on television and radio on programs such as PBS’s Newshour, ABC’s World News Now, NPR’s Science Friday, the History Channel special Clash of the Cavemen and the Science Channel series Space’s Deepest Secrets. John has also been an adjunct professor of science writing at New York University since 2009. Most recently, he was editorial director of McGraw-Hill Education’s online science encyclopedia AccessScience.
Reprinted with permission from Quanta Magazine’s Abstractions blog.