It was much too warm for a Massachusetts mid-October evening, and the bugs were there to say so. A sonic blanket lay over the Alewife Brook Reservation, a 136-acre spread of wetland, forest, and meadow just outside Boston—the buzzsaw purr of cicadas and the katydids’ rhythmic zipper noise woven between the pure-tone chimes of crickets—making it sound like late summer. This sound has felt especially welcome lately, amid reports of dwindling global insect populations usually labeled an “insect apocalypse” in the news.
Despite the racket, I couldn’t see any insects until Avalon Owens, an entomology graduate student at Tufts who has what she calls “super-vision for tiny things,” bent down to point out a sleeping bumblebee, nestled on the underside of a shrub’s leaf. And then another, under another leaf, and then two more. I pointed out a mosquito that had landed on her forehead; she gently brushed it away with a forefinger.
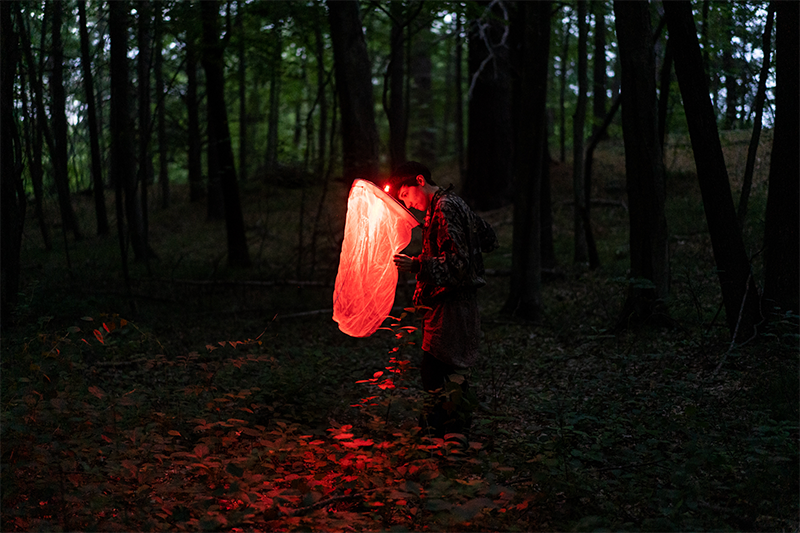
Owens—bumblebee earrings hovering over the collar of a hunting camo fleece—tells me my difficulties in fully perceiving the insect world around me aren’t unique to me. I had come to talk with her about her theory that part of what scientists call a decline in insect population is not an actual decline; rather, over the past few decades, bugs have started to hide where entomologists aren’t looking. Her reasoning goes like this: Since humans have been artificially illuminating the night, they have noticed insects congregating around lights. First there were a few at campfires, then a flutter at gas lamps, then flocks at gas stations and streetlights. Entomologists took advantage: To find insects or count them, they turned on lights. People started using lights to attract insects more than three centuries ago and still today, researchers collect much of their insect population data with light traps at night.
“You put out a light and see what comes to it,” Owens said. “And if they stop coming to it, you’re like, ‘Oh, there’s no insects left.’”
But think about what happens to those bugs, drawn to candle flames or gas stations, or entomologists’ light traps, for that matter. They’re incinerated, or mashed against a headlight, or become a well-lit snack for predators. And even if they survive their encounter with the light, they’ve spent their night loitering instead of eating or finding a mate. Generations of insects later, the ones that remain are more likely to be the great-great-great-grandlarvae of the ones that stayed away.
Why did insects have the “most ruinous propensity” to “rush impetuously into bright flames?”
If Owens is right that insects have been evolving to avoid light, then many of the observations on which some scientists base an insect apocalypse may actually indicate more of an insect-observation error. We could be trying to observe insects by looking exactly where they aren’t.
That would be a problem, because insects are very important. They perform what ecologists call “ecosystem services,” pollinating plants and eating pest species. And while doing that, they move the great drive belt that governs all life on the surface of the Earth: the transfer of energy from the sun to plants to animals to other animals. Insects, weighed all together, would probably be heavier than any other group of terrestrial animals. They achieve this mostly by eating a tremendous amount of plant matter—so much that the food preferences of local insects can determine which trees make up a forest, Laurel Symes, a Cornell ecologist, told me. Their appetite means much of the solar energy in plants has to go through insects before it goes anywhere else, and the rest of the animal kingdom knows that. “Everything will eat an insect if it can get ahold of it,” Symes said. That “everything” includes birds, frogs, spiders, small mammals, some other insects, and Yellowstone grizzly bears, which can eat up to 40,000 army cutworm moths in a day, scooping them by the pawful from rocky crevices.
My first reaction to hearing Owens’ hypothesis was relief—a feeling that, if reports of the “insect apocalypse” had been based on faulty methods, then maybe that was at least one less thing to worry about in a world full of other, more dependable apocalypses. But unfortunately, Owens isn’t saying that everything is just fine with insects and their ecologies. Global apocalypse or not, there’s a lot of compelling evidence that insect populations of some species are declining and changing in some areas, especially those affected by human activities such as agriculture, which are also some of the places insects are most important to us. There is broad consensus among entomologists and ecologists that we should at least be worried about these species and the broader ecological effects of the changes in their numbers.
So if one of our main methods for finding and studying insects turns out not to work, where does that leave us? We need to understand insects, and if Owens is right, we don’t understand them at all.
Among the things we don’t understand about insects is why they would come to light in the first place. I had always assumed it’s just a thing that insects do, but hearing from Owens about the terrible dangers insects brave to buzz around lights made me think again. Of course, I am not the first to wonder.
In April 1871, South African butterfly and moth naturalist Roland Trimen wrote to ask Charles Darwin my very question: Why did insects have the “most ruinous propensity” to “rush impetuously into bright flames?” The behavior could only have developed recently, he guessed, “after man began to lighten his darkness by artificial means.”
Trimen also offered a tentative prediction based on Darwin’s theory of evolution: “the elimination by death or severe injury of all those individuals in whom the propensity was strongest, might eventually rid a species of so peculiar a form of suicide.”
Was it possible that insects had started to shake their fatal attraction to lights?
Almost a century and a half later, in summer 2010, Dieter Ebert was sitting on the porch of a remote field station in rural Finland contemplating the same question. Ebert, an evolutionary biologist at the Zoological Institute of the University of Basel in Switzerland, and his graduate student Florian Altermatt were there to study crustaceans. But every evening they would sit on their back porch and watch the moths flapping around the lights there.
“There was always this question,” Ebert said. “Why do they fly to the light?”
Darwin hadn’t been able to answer Trimen’s question, and it still doesn’t have any universally accepted answer. Ebert’s favorite hypothesis, he told me, is the so-called “moon compass theory,” that insects have evolved to navigate by keeping a constant angle to the light of the moon, but trying to keep a constant bearing with respect to a light only feet away leads them to spiral into it.
Pondering the moths in Finland, Ebert remembered a white wall behind the porch of the farmhouse in Western Germany where he had grown up in the 1970s. There was a light in the middle of it that his family turned on at night, and Ebert would sit out and watch hundreds of moths land on the wall, until it was fully covered.
Ebert told me that in 2010, it seemed to him he didn’t see as many moths as he had back then.
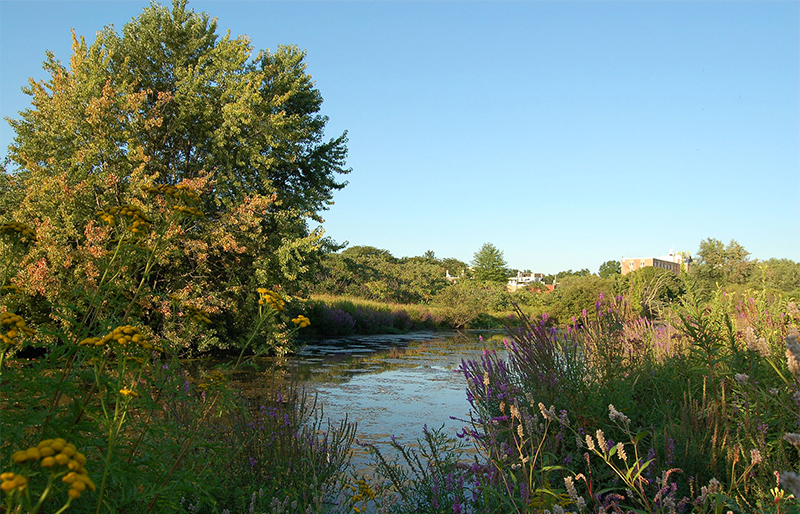
Forty years might not seem like much time on the scale of natural history. But evolutionary time is measured in generations rather than in years, and there is often more than one insect generation per year. Ebert specializes in studying cases of “fast evolutionary processes,” like a population of Swiss water fleas, a crustacean that can evolve immunity to a deadly bacterium over the course of just two months.
“I have seen many cases where things evolve quite fast,” Ebert said. “And once you understand that selection is strong, undoubtedly you will find the effect of adapting in response to the selection.”
And in the case of insects, he said, the selective pressure of artificial lights on those that come to them is “extremely strong.”
So he and Altermatt began to wonder: Was it possible that in the best-lit places insects had started to shake their fatal attraction to lights?
One of the best already-confirmed examples of fast evolution happens in insects. Over the course of the 20th and 21st centuries, more than 500 species of insects have evolved a resistance to specific pesticides. On Ebert’s principle, that makes sense: A chemical tailor-made to kill you is a very strong selective pressure.
But millennia before people started to produce pesticides on an industrial scale in the 20th century, they figured out another cheap way to dispatch insects in bulk: to exploit their attraction to light. Ancient Greek and Roman beekeepers were already using open fires to attract and kill the wax moths that colonized their hives, and the method persisted until the 1860s when Townend Glover, the first United States Entomologist in the Department of Agriculture, was recommending “bonfires in June” as a method of controlling codling moths, whose larvae like to live in apples, pears, and English walnuts. Still today, modern light traps are an important tool for pest control.
At the same time people were exploiting insects’ weird but useful attraction to light to kill them, another group began to use it to study them: entomologists, who worked alongside insect-killers over the years to devise ever more effective ways to trap insects.
In the 1860s, Glover (of the bonfires in June) invented the first version of the self-contained light trap—an assemblage of glass panes, leather straps, sliding drawers, and a kerosene lamp. You could set the “marvellous moth trap” out for the night and come back in the morning to study your catch (a welcome improvement over the method of wandering the woods at night with a candle, as entomologists had been doing for the previous few centuries).
Today, much of what we (“we” as in entomologists) know about insect populations comes from those sorts of traps (albeit updated versions with electric light sources). One blockbuster “insect apocalypse” article in 2019, for example, was a review of previous insect decline papers, and described “dramatic rates of decline that may lead to the extinction of 40% of the world’s insect species over the next few decades.”1
There are some technical issues with the 2019 review, like the extrapolation of population decline in certain areas to a worldwide loss of insects (this seems like a stretch to many ecologists, including Ebert and Owens).
But also, many of the papers the 2019 review cites use data from light traps. The papers it uses to assess United Kingdom moth decline, for example, rely heavily on the Rothamsted Insect Survey, one of the oldest continuous insect population observatories. Since the 1960s, the survey has run two trap networks in the British Isles, one of which monitors moths with light traps, and the other of which monitors aphids using suction traps, which suck in flying insects passing by. In 2020, it published a result that might not surprise you if you’re inclined to share Owens’ suspicions: While it found moth populations appeared to have declined by about 31 percent over the past half-century, aphid populations had remained just about stable.2
From an insect’s perspective, the light problem is much bigger than scattered bonfires and entomologists’ lanterns and bulbs. What matters to an insect is that in the last 100 years or so, much of the world’s nighttime has become much brighter—a process that has dramatically accelerated in the last 15 years with the widespread adoption of LED lighting. Illuminating the night is now easy, cheap, and (with respect to carbon emissions) relatively environmentally friendly.
These days, it’s hard to find a spot in the United States where you can see the Milky Way at night. A light is no longer such a “very new object” to insects as it had been to the ones Darwin considered in 1871.
So in Finland in 2010, it seemed reasonable to Ebert and Altermatt that the insect populations most subjected to lights would have already begun to evolve away from their attraction to them. They devised an experiment to find out: They would check if urban moths were less likely than rural moths to come to a light.
They set about collecting spindle ermine moth caterpillars—small white caterpillars with black spots, which turn into small black-speckled white moths—from the French and Swiss countryside, and from parks and bushes near city street lights.
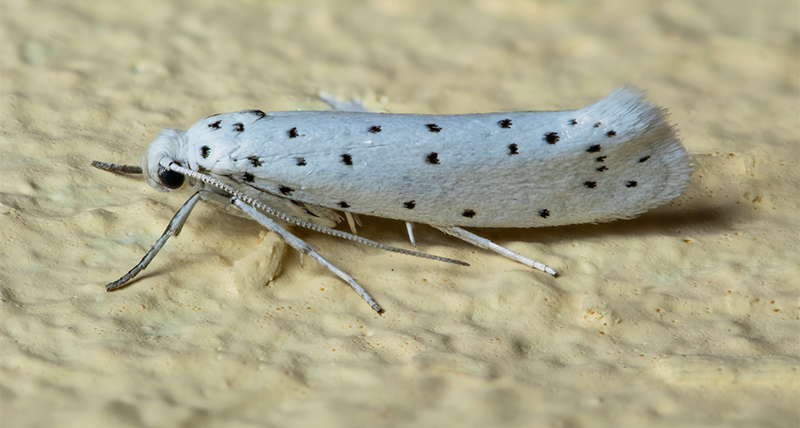
After a caterpillar hatched into a moth, the researchers would dye a dot on one wing, tagging it as a country moth or a city moth. Then, in the evening, they took it and its cohort of hatchlings into a room completely tapestried in black fabric. They set up a light trap at one end of the room, released the moths at the other end, and waited.
The work of coming back to the lab in the middle of the night, to check on the moths and record the ones that had been trapped and the ones sitting on the walls near the light, mostly fell to Altermatt. But Ebert remembers that on the one night he came in, it seemed like about equal numbers of city and country moths had come to the light.
As they started to crunch their numbers though, a pattern emerged. About 30 percent fewer of the city moths appeared to be attracted to the light.3
That’s not a “super strong” effect, Ebert said. That could be in part because in some city bushes they had unwittingly collected the caterpillars of moths that had flown in from the country. But it could also be because the process of evolution away from light is an ongoing one.
“It might well be that if you do the same study in 20 years, that the effect is stronger,” Ebert said.
But the effects of moths’ evolution away from light-seeking behavior could go beyond a divide between urban and rural moths, Ebert added. The more advantageous it becomes to not be attracted to light in the city, the likelier that behavior will be to spread through the whole species.
And today, Owens believes that the widespread loss of light-seeking behavior, across many species of insects living everywhere from cities to the countryside to rainforests, may have already begun.
On our walk through the Alewife Brook Reservation, Owens crouched down to point out a recent adaptation: A spider had strung its web across a light illuminating the walkway. “Evolution in action,” she said. If spiders have learned to come to lights to catch bugs, why shouldn’t bugs have learned to stay away from lights and avoid being caught by spiders?
Owens’ evidence, which she will use to investigate her hypothesis on a postdoctoral fellowship at Harvard, will be moths, many of them pinned in drawers in Harvard’s Museum of Comparative Zoology.
“We’d have no idea if we had half as many insects now as we had 10 years ago.”
Owens will use those moths, as well as data about captured moths in other collections, to answer two questions: How has the likelihood of entomologists capturing a given species of moth with a light trap changed over the past decades? And, how have the actual population sizes of that species varied over the same time period?
“You have two lines,” Owens told me. “One line is what we think is happening to insects based on light trap data, and one line is what’s actually happening to insects based on actual estimated population size.” The plausibility of her hypothesis will be determined by the discrepancy between the two lines.
To find the shape of the actual-population line, Owens plans to sequence the genes of historical moth specimens in the MCZ collections from different years, and use changes in gene diversity between specimens as a proxy for changes in population size (when populations of a species are smaller, individuals are also generally more genetically similar to each other). And for the observed-at-light-trap line, she will need to determine how, and how often, entomologists caught what they caught, using whatever records they happened to leave behind.
In Harvard’s lepidoptera room, I began to see why that second task is so difficult. Much of the data about how a given moth was collected exists only as handwritten one-inch-by-one-quarter-inch note cards pinned under the moth, or worse, in the field notes of whichever researcher collected it. Often, especially for older samples, researchers don’t mention their methods at all. And even if a researcher collected a moth with a light trap and recorded that, and the information was later entered into a museum’s database—each step less likely than the last—unless they were one of a handful of completely systematic surveyors they might have only preserved those species that they happened to be interested in.
Beyond those issues, studying insect populations is just a data science nightmare. This is partly a problem of scale: About four-fifths of all documented animal species are insects. And although more than a million species of insect have already been discovered, there are likely millions more out there waiting to be found.
The number of individual insects also tends to change wildly year-by-year, in “big booms and busts,” Owens told me. Although some of those correspond to events like temperature changes and droughts, “a lot of it is just literal, chaotic noise,” she said.
More fundamentally, trying to understand insects also poses problems for humans as an animal species. Namely, “lots of insects are invisible,” Symes, the Cornell ecologist, told me. They are just much better at hiding than we are at finding them. So, humans haven’t yet been able to understand anything about insect populations with much precision.
“If you have a year-over-year 10 percent decline for 15 or 20 years, A, it matters a lot; and B, it’s crazy how that could happen and we really wouldn’t notice it,” Symes said. “We’d have no idea if we had half as many insects now as we had 10 years ago.”
To an insect, if you’re not a potential mate there’s a high chance you’re a predator, so insects really don’t want you to find them. But if you are a suitor, they need you to. The result: “a lot of insects are nearly invisible, but they’re not all silent,” Symes said.
Often, insects communicate with each other from their hiding spots with chirps and buzzes. And to find them, she said, “we basically have to talk on their channel, or listen on their channel.”
Even once you’re listening on their channel though, insects can still be frustrating to get ahold of. “Tree crickets are surprisingly hard,” Symes told me. “My first field season was almost entirely learning how to catch crickets.” The trick, if there is one, seems to be in a combination of detailed anatomical knowledge of your prey, a good ear, trigonometry, and calisthenics.
“Because of the wing structure of tree crickets, the sound goes out in front and behind with almost nothing that goes to the sides,” Symes said. “You’ll lose the sound you’re searching for sometimes, unless you have a really clear mental image of that shape. And then if you’re able to walk around the space where they’re hiding, you’ll hear, ‘Loud, quiet, loud … Okay, it must be somewhere in between these two loud points.’ And then it involves a lot of standing up and crouching down to be like, ‘Okay, this is the height where it’s the loudest.’”
And after all that (and assuming you’re able to catch it), you’ve got yourself one cricket.
As a human, you don’t really notice how much of the night is dominated by electric lights, until you do.
Entomologists trying to observe the insect world have generally shared the goal of capturing insects with insect predators. That’s inconvenient for entomologists because it’s a goal insects have spent their whole evolutionary history learning how to thwart. Anything an entomologist does that ends in an insect’s capture is by definition a selective pressure on the insect, and in many cases imitates other selective pressures that are very present in the insect’s life. The insect responds by pretending to be a leaf, or, possibly now, by learning to stay away from light bulbs.
To Symes, the solution to that problem is to observe insects without touching them, or even seeing them. She is the assistant director of the Center for Conservation Bioacoustics at the Cornell Lab of Ornithology—a center of which the guiding philosophy, essentially, is to “listen on their channel.” Symes’ work involves climbing into Central American rainforest canopies, leaving sound recorders there for months to capture choruses of singing insects, and then digging through the recordings for information about the singers’ population sizes, mating habits, territorial grumpiness, wake-up times and bedtimes, and anything else a bug reveals when it talks.
“If you walk out there and listen, all of a sudden you have a much richer picture of what’s in that space,” Symes said. “I don’t know exactly where this insect is sitting, but I know that it’s there, whereas a predator actually has to catch it and eat it.”
While a predator (or an entomologist) might get tired of trying to track down a hidden and intermittently-singing insect and move on to an easier meal, other insects have all night to slowly detect and crawl toward a mate. Symes’ way of observing insects—a technique called Passive Acoustic Monitoring, or PAM—seems more like how an insect would go about it. When Symes takes a long-term recording and then spends hours listening to and annotating it in the lab, it’s a sort of technological mimicry of that insectine patience.
Symes’ access to the insect’s-ear view (actually, insect’s-knee view in the case of crickets and katydids, whose ears are on their legs) yields questions about insect lifestyles that wouldn’t have been askable without it. For example, she noticed in a set of recordings that one species of katydid only chirped in the pre-dawn hours.
“The interesting thing is they’re one of the only brightly colored species,” Symes said. “So I don’t know if they maybe meet up right before dawn and then take a look at each other when the sun comes up and decide whether to mate or not—I’m not sure what unique use they’re making of that time slot.”
This mode of insect observation, freed from the human need to understand by capturing, collecting, and pinning on a board, also allows the Center to collect data on whole populations of singing insects at once. “If you get 50 calls per hour of a species this year, and next year you get 200 calls per hour, or 10 calls per hour, that’s telling you something,” Symes said.
That “something,” she hopes, will be a better understanding of the species’ population size, but it could go beyond that. The PAM technique is relatively recent, and the first long recordings the Center has are from 2016. As they collect more data, they’ll be able to draw new inferences. You might record more calls from one species during a particularly wet season, or fewer during a dry one, Symes said. “You start to get some mapping between what the insects are doing and what the environment is doing.”
The channel on which Owens talks with and listens to insects, for the most part, isn’t sound. She talks (and even flirts) with them using light. To experiment on male firefly mate preference, for example, she programmed an LED “firefly communicator” to flash like a female firefly.
It was Owens’ observations of fireflies that led her to be thinking about light pollution, which in turn helped her come up with her hypothesis about insects evolving to no longer seek light. Light pollution is especially traumatic for fireflies, which need to be able to distinguish each others’ flashes from, say, a semi’s headlights, to reproduce. Owens has spent the past few years documenting the light-pollution-driven decimation of American firefly populations.
Light pollution isn’t just destructive to fireflies. We, and many other species, rely on melatonin, a light-responsive hormone, to regulate our sleep cycles—a mechanism so evolutionarily ancient a version of it is present in plants. On that time scale, a light is still a very new object. Nighttime lights have been shown to disrupt, among others, migratory birds’ flight patterns, frogs’ and toads’ mating, and mice’s foraging. And now, with the widespread adoption of LED lighting, the night is brighter than ever.
As Owens got up from looking at the bees, curled up under their leaves for the night, she pointed out a new biomedical research facility looming up just outside the reservation.
“It’s green architecture, and it’s super eco-friendly, and it’s right next to the reserve,” she said. “And isn’t that nice, humans and nature living together?”
To avoid the energy costs of heating and absorb as much sunlight as possible, the building was equipped with huge bay windows. And all night, energy-cheap LED lights blaze out of every floor and out into the reservation. I imagined the sleepy bumblebees bumbling off deeper into the woods, rudely stirred up by the city’s accidental floodlights.
As a human, you don’t really notice how much of the nighttime visual landscape is dominated by electric lights, until you do. Walking out of the reservation after dark, the lights felt visually aggressive—blinding splotches of white and orange blotting out the night around them. Suddenly, I wanted to see what the lights were hiding.
I looked at the lights around me, shining out of the Alewife Station parking lot, skimming across the reservation’s creek and marshland. The insect song was everywhere but I still couldn’t see any insects, even looking up at the streetlamps’ globes.
“I don’t see anything,” I said, pointing, feeling smart.
“Yeah…” Owens said. “It’s also October,” she cautioned. She kept looking.
“Oh, there’s one!” She pointed at the lamp and I saw a flicker of wings. “Actually, there’s a few.” Following her finger, I could barely see a small troupe of flying insects, dancing around the periphery of the lamp’s glow.
Oliver Riskin-Kutz is a student at Harvard, studying environmental history and biology. He’s interested in the ways humans and other kinds of life live with and around each other. His writing has appeared in Berkeleyside and the Harvard Crimson. You can follow him on Twitter @OLRiskinKutz.
Lead image: Sandusit Noom / Shutterstock
References
1. Sánchez-Bayo, F. & Wyckhuys, K.A.G. Worldwide decline of the entomofauna: A review of its drivers. Biological Conservation 232, 8-27 (2019).
2. Rothamstead Research. British moths declining but aphid numbers stable. www.rothamsted.ac.uk/news (2020).
3. Altermatt, F. & Ebert, D. Reduced flight-to-light behaviour of moth populations exposed to long-term urban light pollution. Biology Letters 12, 20160111 (2016).