Atlas knew the answer. Straining under the task of holding up the Earth, this Titan god likely got a good idea of how much the Earth weighed. But none of us are so conveniently situated. How could a mere mortal, a tiny person residing on Earth’s surface, carry out their own estimate of Earth’s weight? Where would—where could—you possibly place the scale?
A firm answer didn’t arrive until the Englishman John Michell figured out a way. Little known today, he was actually one of the cleverest clergymen of the 18th century. As a geologist, astronomer, mathematician, and theorist who hobnobbed with his fellow greats at the Royal Society of London, he was the first to many things: the first to suggest that earthquakes propagate as elastic waves through the Earth’s crust (earning him the title “father of modern seismology”), the first to suggest that many stars were likely paired up as binaries, and the first to imagine a star so huge and massive that no light could escape its intense gravitational field—a black sun, the Model-T version of a black hole.
This West Yorkshire parson was a devotee of Isaac Newton’s law of gravitation, introduced in 1687. While the law had found great success in predicting the motions of comets and cannon balls, by the 1780s the gravitational attraction between two small bodies in a laboratory had not yet been demonstrated, according to Russell McCormmach, author of the 2011 book Weighing the World. With his longstanding work in geology, Michell had wanted to know for decades our planet’s density—and hence its weight. So, he devised a scheme to measure those short-range gravitational forces, and in the process weigh the Earth. The apparatus Michell designed was not complex, yet elegant. In fact, it involved only four balls of lead, a moveable rod, and a set of wires, all encased to prevent interfering air currents. Physicists call this a “torsion balance,” as rotation of the rod is the key to its function.
A delicate balance leads to a colossal result.
The final design had a six-foot-long wooden rod suspended on a wire, with a pair of the two-inch-wide balls attached to each end. Then a larger ball (a foot across) was separately suspended close to each of the smaller balls. The idea was that the minuscule gravitational attraction between each pair of large and small balls would gradually cause the supporting arm to move. But this rotation stops once the twisting force of the wire equals (in other words, is balanced by) the force of attraction between the large and small lead spheres. This provides one bit of information. Already known is the gravitational force of the balls toward the Earth below; it’s simply their weight. Crucial to Michell’s concept was having these two sets of data. By comparing the two, separately measured forces on the balls, the experimenter can then calculate the one unknown in the gravitational equation—Earth’s mass. A delicate balance leads to a colossal result.
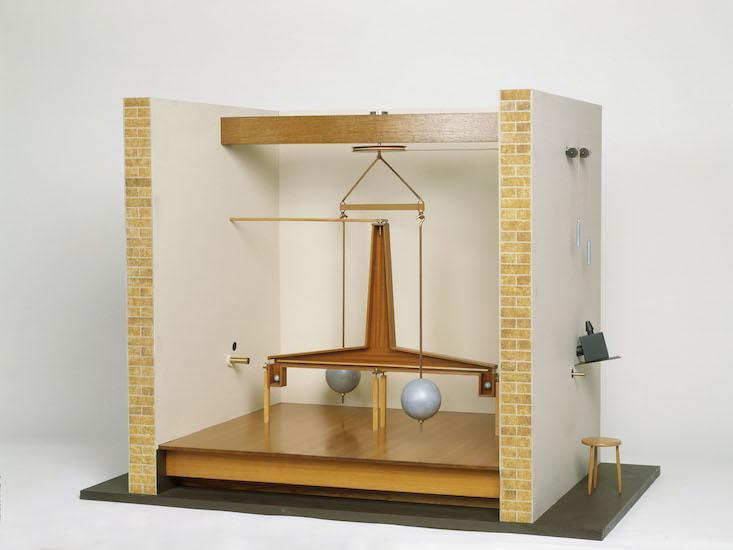
This experiment, however, was difficult to build and operate. In 1784, Michell had written his Royal Society colleague Henry Cavendish (discoverer of hydrogen) that he hoped to weigh the world “in the course of the Summer.” But both ill health and “natural indolence,” as Michell put it, prevented him from ever completing his project. He was also distracted by trying to build one of the largest telescopes ever. By his death in 1793 at the age of 68, he had never carried out a measurement.
Michell’s apparatus eventually ended up with Cavendish, who was described by his biographers as “one of the richest men of the realm…a scientific fanatic, and a neurotic of the first order.” He was frightfully shy, especially of women. In his solitude, he completed the instrument with the final design that improved on Michell’s original scheme. Cavendish now gets the lion’s share of credit for the long-awaited test—and with good reason. With the apparatus shut up in a small shed on his estate, he had to manipulate the weights from the outside with pulleys and observe the arm’s tiny movements (no more than 2/10th of an inch) through a hole at each end of the shed’s wall with a telescope.
It was laborious and painstaking work. Over and over again, he measured torques, moments of inertia, and the angles of the rod’s deflections, with the results inserted by hand into the proper mathematical formulas to find answers. His discovery paper, published in Philosophical Transactions in 1798, was described by a Scottish physicist at the time as “a model of precision, lucidity, and conciseness.” Cavendish’s computed value for the density of the Earth—even with equipment we now consider ancient—was within one percent of today’s value, now recognized as 5.513 grams/cm3, or five-and-a-half times the density of water. Multiply that by the volume of the Earth (around 1.1×1027 cm3), and you arrive at some six thousand trillion trillion grams for our planet’s total mass.
Today scientists continue to carry out this classic Cavendish test, although with quite different methods and with a different aim in mind. They seek more precise measurements of the gravitational constant G, a fundamental factor in Newton’s universal law of gravitation F = G{(m1m2)/r2} that relates force to mass and distance. This quantity is less settled than other fundamental constants in physics and more accuracy is vital “because of the key role that G has in theories of gravitation, cosmology, particle physics and astrophysics and in geophysical models,” wrote Guglielmo Tino, a physicist at the University of Florence, and colleagues, in a 2014 paper.
His team, from Italy and the Netherlands, conducted the experiment “using,” they wrote, “laser-cooled atoms and quantum interferometry.” In other words, by measuring the gravitational force between a cloud of rubidium atoms and heavy tungsten cylinders, they were able to determine the value of G as 6.67191×10-11m3kg-1s-2 with a relative uncertainty of 150 parts per million. That gravity is the weakest of nature’s four forces is aptly demonstrated in that tiny number. Too bad Atlas isn’t around these days to confirm the finding.
Marcia Bartusiak is a professor of science writing at the Massachusetts Institute of Technology and the author of six books on astronomy and astrophysics. A new edition of her classic work on gravitational-wave astronomy, Einstein’s Unfinished Symphony, will be published this June.
WATCH: The physicist and writer Alan Lightman on the moment of his first discovery.
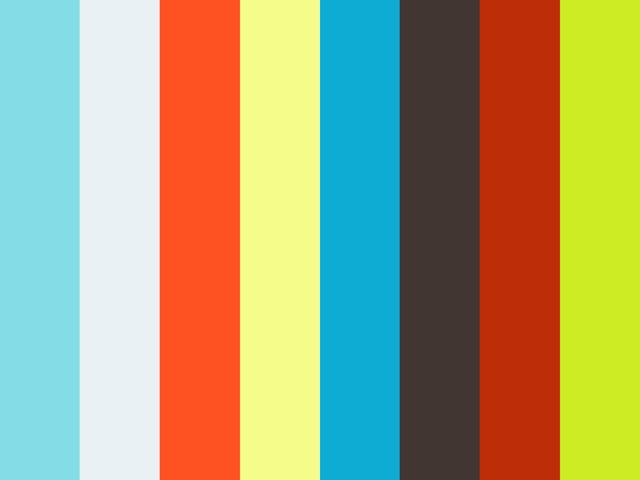