As the rains return to the Pacific Northwest, so do the mushrooms. Fine mist drips from the crowns of evergreens, through the mosses and down into a layer of fragrant duff that shelters an ecosystem of mycelium, bacterial decomposers, and insect aerators. Under the canopy of old-growth forests, everything is dying, being taken apart at the molecular level—but at the same time, it is being reborn. A fallen tree becomes a nurse log, home to organisms that nurture new forest life. It’s a landscape of cataclysm and of regrowth, where nature shows off its singular ability to recover and revive, year after year.
The environmentalist movement that was cultivated here has given rise to an entire generation of engineers, scientists, and designers who have started to pay closer attention to the ways that nature can be the most sagacious teacher. Mycologist Paul Stamets, for one, has shown that a mycelium extract can decompose petroleum waste and sprout oyster mushrooms in its place. These visionary scientists are leaders in the biomimicry movement, in which scientists are harnessing nature’s processes to right our environmental wrongs. Featured below are five current examples of biomimicry seeding a sustainable future.
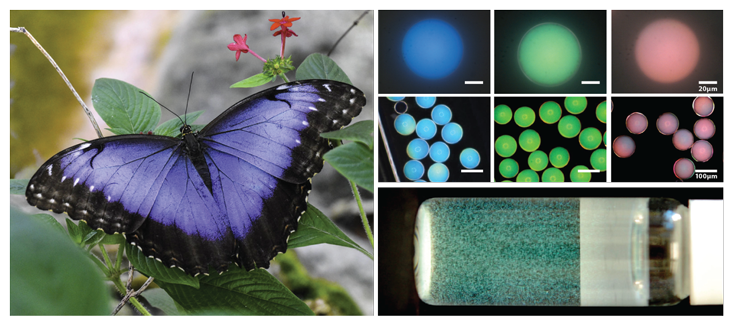
Colloidal Pigments
Making the vibrant dyes we use for everything from house paints to fabric to cars is a toxic, energy-intensive process. The very property that creates the most satisfying, long-lasting colors is exactly the resonant, high-energy behavior that makes them so deadly. Phthalo green, chrome yellow, cadmium red are lovely, but they come with a pernicious price—all are composed of heavy metals poisonous to humans in varying degrees. Many members of the natural world, though, appear in a dizzying array of hues, all while avoiding the pitfalls of heavy metals. How do they do it, you might ask; can’t we just do what they do?
Most biological pigments aren’t synthesized from scratch—they’re usually borrowed from someone else. The soft pink of salmon comes from a diet of coral-shelled shrimp and krill. The bright red plumage of cardinals? From the carotenoid-rich diet of the most succulent forest berries. The color emanates from these creatures on the molecular level, and isn’t very long-lasting. Its stability requires constant renewal. In search of more permanence, material scientists have been looking to more ingrained biological colors for inspiration.
For some reason, many blue creatures in nature have a structural approach to color, rather than the molecular approach outlined above. Take, for example, the opalescent wings of a morpho butterfly. Though they appear an electric blue, the wings themselves are colorless in the traditional sense. There’s no vibrating molecule of blue pigment. Instead, the color comes from the organization of the butterfly’s surface. Each magnificent wing is covered in billions of tiny filaments, each a tenth of the width of a human hair. These colorless structures capture light like the inside of a kaleidoscope, reflecting and refracting it until it comes back that luminous indigo. The structural color of the morpho wings is angle dependent, though—it shimmers and changes as the butterfly flutters. For something more stable, researchers looked to the noble bluebird.
“The colors of nearly every blue bird that you see in nature comes from nano-pores in the feathers, and not from any pigment,” says Vinothan Manoharan, head of a group at Harvard that studies how to create structural colors. These pores are scattered across the surface of the feathers, “disordered in the way that they would be, for example, if you took a bunch of ball bearings and threw them into a bucket without doing any arranging.”
This means that, using their methods, it’s theoretically possible to produce cosmetics, posters, and inks that are completely free of heavy-metal pigments. Widespread use of this technique could lead to a world where object and color are, quite literally, inseparable.
Evaporation Energy
In nature, any spontaneous flow between two reservoirs can be harvested to produce power. Place a water wheel in a river, a windmill on a naked hilltop, attach some magnets, connect a circuit, and you’ve got yourself some electricity. As humans cast about for ways to power our existence without tracking so much mud through nature’s spotless temple, there’s one approach that has been largely overlooked—evaporation.
In some ways, it makes sense; evaporation is such a quiet process. One could be forgiven for barely noticing it—a waft of steam from a cup of coffee on a crisp, fall morning, or mist rising from the roof after a sudden summer downpour—it’s more the domain of poets than the rest of us. But about a decade ago, Ozgur Sahin of Columbia University was studying the mechanical properties of bacterial spores when he was shot through with a bolt from the blue.
His measurements astounded him—the force the spores generated as they cycled between small and thirsty and massive and moist was enough to act like a powerful, humidity-controlled muscle. He still remembers the awe he felt when he realized what was happening. “These tiny soil-dwelling organisms had been carrying this potential all this time and had gone unnoticed,” he recounts. The next stage was to create some way to demonstrate the untapped promise—machines that could run on pure water.
“It took several years and the help of brilliant students and colleagues to make those engines a reality,” Sahin explains, but eventually, his team created a functional prototype. It was a small box filled with spore muscles—thin strips of plastic coated on one side with a spore mixture—that straddled the air-water interface. When the cycle starts, the shutters on the top of the box are closed, which allows the reservoir that contains the muscle to fill with water vapor. The moisture causes the muscles to relax, which opens the shutters. As the water evaporates from the spores the muscles contract, which resets the cycle.
As the muscles cycle through wet, dry, wet, dry, their motion turns an electromagnetic generator, as long as there is water in the reservoir. A former colleague of Sahin’s, Xi Chen, is iterating on nature in an attempt to produce an artificial protein that is even more water-responsive than the shell of the original bacterial spores.
This method, if scaled up, could be extraordinarily effective considering that almost 90 percent of the atmospheric moisture is the result of evaporation from air-water interfaces. The team believes their technology can match existing green-energy alternatives, but without the intermittency issues of solar and wind.
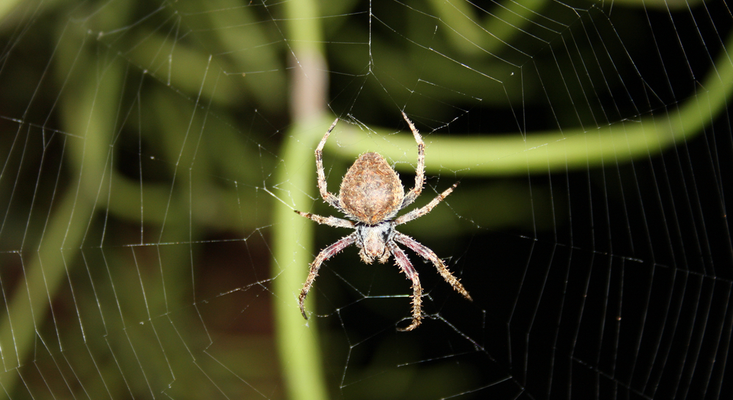
Spider Silk
Spider silk “is arguably nature’s toughest material, and has other properties like biocompatibility and electro-thermal conductivity,” explains Sean Blamires, the principal investigator leading University of New South Wales’ Spider Silk Research Laboratory. The extraordinary material has a host of properties that make it well-suited for a variety of applications. Its surface chemistry makes it ideal for use as a biocompatible coating for implants, one which lowers the risk of rejection while it accelerates healing. Its supreme strength makes it ideal for artificial muscle fibers in next-generation prosthetics, and its biodegradable nature makes it an excellent option for packaging, so we can finally stop wasting the most durable materials on earth for the most temporary task.
In addition to the space-age applications, there’s a more prosaic vision of a smoother future—where biologically-based strength, elasticity, and toughness make spider silk an extremely attractive alternative to man-made fibers like rayon. Although rayon technically comes from recycled wood products, the process of manufacturing industrial quantities is stunningly dirty. To extract usable fibers from wood pulp, workers have to apply a sequential mix of caustic chemicals, and are exposed along the way to carbon disulfide, a volatile byproduct that can cause everything from priapism to death by respiratory failure.
Synthetic spider silk solves all of these problems. Engineered bacteria produce spidroins, the component proteins of spider silk. These proteins are then purified, concentrated, and resuspended in water. When the protein mixture is squeezed through a tapered syringe into a saline solution, it results in clean, spinnable fiber that can be degraded at the end of its life by naturally-occurring enzymes. This unrivaled sustainability has attracted the attention of high-profile designers like Stella McCartney, who has partnered with a company called Bolt Threads to produce some of the world’s first knit hats and tennis dresses made from engineered spider silk.
Blamires, though, is less interested in the newest tennis dress than he is in understanding why spider silk is so uniquely stiff, tensile, and tough. His group is working on a survey of silk production in different spiders, across a variety of ecosystems. They hope this will help them get a better grasp on the evolution of silk’s coveted properties. “We think that knowing the natural silk production processes and its variability therein is very important. We should look at what nature does and try to imitate it,” he concludes. Which is just fine, as long as he remembers to make sure that the big room, full of big spiders, is safely locked before he goes home for the night.
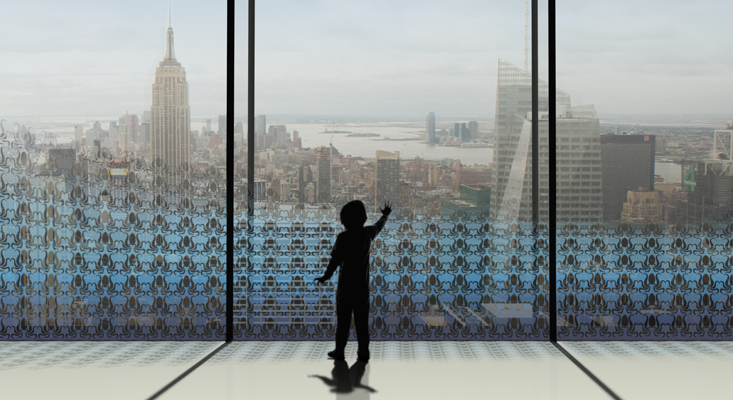
eSkins
Urban centers are well known to be “heat islands,” places where temperatures are several degrees hotter than in the surrounding countryside—a side effect of the ways that we’ve been building cities for the last few centuries. Roads are dark, flat surfaces that absorb an inordinate amount of heat. Buildings, especially skyscrapers, have their own heat peaks—all those central cooling towers and countless window AC units just dump the hot air onto the already-sweltering pavement.
For Shu Yang, professor of material science at the University of Pennsylvania and director of the Center for Analyzing Evolved Structures as Optimized Products, the answer to this urban heat problem is biomimetic design on the city scale. Yang’s work focuses on the fundamental concept of homeostasis, informed by her collaborations with biologists. She notes that her team “sees a unique functionality, and then looks to biology” for instruction on how to approach the problem. In her world, insight into nature starts on the scale of miniature and is transmuted to the monumental.
Take, for example, the problem of office buildings. Most older offices spend almost 40 percent of their electrical budget on heating and cooling. It’s usually a centralized process that’s insensitive to minute changes in the environment. A corner office in direct sunlight is sweltering, while the conference room under the eaves of the building always requires a sweater. Yang is interested in the idea that if buildings could be more like animals—if they had a way of sensing their environments—maybe they could change their behavior in order to be more habitable.
To achieve this, Yang’s team combines the biological with the structural. Intrigued by the chromatic structures in cephalopod skin—small pigment sacs that allow creatures to go from transparent to opaque at the slightest sign of danger—her team developed a material with similar properties that could be used to finely tune building conditions. It can be added onto existing buildings like a second skin that’s completely transparent when relaxed, but becomes opaque when stretched.
In the future, she imagines merging this new material with kirigami design, where applied tension can create complex, three-dimensional shapes. This could produce building skins where “one side is reflective, but you don’t normally see the reflection. When it starts to get hot, we can tilt the panels to reflect the light.” She imagines that these skins could be entirely autonomous if merged with materials that respond to humidity changes—maybe those spore muscles mentioned earlier?
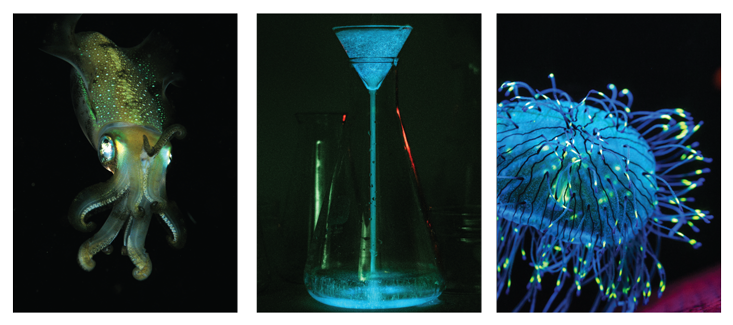
Bioluminescent Cities
Last but not least, bioinspired design may be the most enchanting way to address widespread light pollution.
A little more than 100 years ago, inhabitants of even the largest cities would have been able to look at the night sky and get some sense of their cosmic context—but with increasing illumination, and the brightness of the newest generation of LED bulbs, many are worried that we may be headed for a future where the night sky is little more than a distant memory.
The future, though, could be a little more green—blue-green, to be specific. Inspired by the bioluminescent light produced by marine animals, Glowee CEO Sandra Rey has designed a lighting system that is literally alive.
As Rey recently told Bloomberg, “in the sea something that is very common is not extraordinary—and so you have to understand that if it’s common it means that it is efficient. Behind us we have 3.8 billion years of research and development.”
In the lab, her team seeks out bacteria with the most powerful, long-lived, and stable bioluminescence. They grow these creatures in massive quantities, to crowded conditions which causes the bacteria to activate light-releasing pathways. Under standard conditions, the bacterial soup is a golden color, similar to a patch of yellow brick road. But when the lights go down and oxygen is bubbled through the stock-still, mustard-colored medium, something magical happens. The liquid swirls, sparkles, and suddenly catches into a phosphorescent glow. The result is a stable, peaceful blue light that lends its surroundings the feeling of wandering through an enchanted forest.
In the future, Rey imagines Glowee’s living lamps as the default option. The company plans to start from urban furniture and signage—but with time, hopes to rethink the whole way we see our cities at night. With this achievement, humans may quite literally see themselves in a new light.
Michael Shilo DeLay and Anastasia Bendebury are biologists and co-creators of the science blog, Demystifying Science. Follow them on Twitter @Demystifysci.
Lead image: Ondrej Prosicky / Shutterstock