It’s hard to tell precisely how big a role biotechnology plays in our economy, because it infiltrates so many parts of it. Genetically modified organisms such as microbes and plants now create medicine, food, fuel, and even fabrics. Recently, Robert Carlson, of the biotech firm Biodesic and the investment firm Bioeconomy Capital, decided to run the numbers and ended up with an eye-popping estimate. He concluded that in 2012, the last year for which good data are available, revenues from biotechnology in the United States alone were over $324 billion.
“If we talk about mining or several manufacturing sectors, biotech is bigger than those,” said Carlson. “I don’t think people appreciate that.”
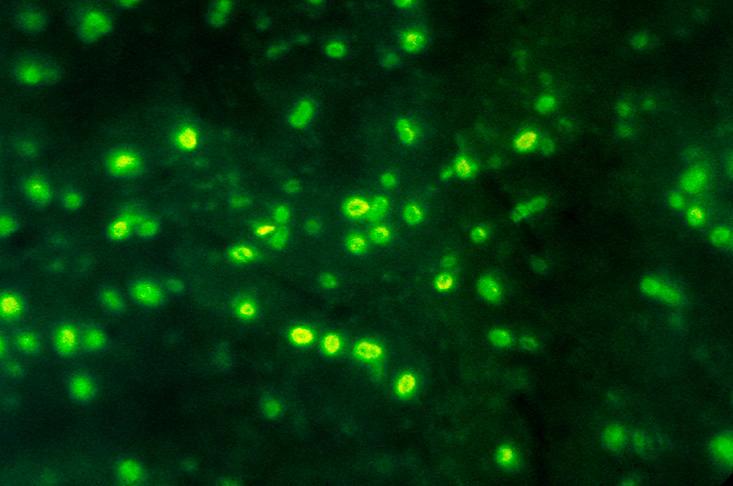
What makes the scope of biotech so staggering is not just its size, but its youth. Manufacturing first exploded in the Industrial Revolution of the 19th century. But biotech is only about 40 years old. It burst into existence thanks largely to a discovery made in the late 1960s by Hamilton Smith, a microbiologist then at Johns Hopkins University, and his colleagues, that a protein called a restriction enzyme can slice DNA. Once Smith showed the world how restriction enzymes work, other scientists began using them as tools to alter genes.
“And once you have the ability to start to manipulate the world with those tools,” said Carlson, “the world opens up.”
The story of restriction enzymes is a textbook example of how basic research can ultimately have a huge impact on society. Smith had no such grand ambitions when he started his work. He just wanted to do some science. “I was just having a lot of fun, learning as I went,” Smith, now 85, said.
If restriction enzymes went on an unchecked rampage, they could kill the bacteria themselves by chopping up their own DNA.
In 1968, when Smith was a new assistant professor at Johns Hopkins University, he became curious about how cells cut DNA into pieces and shuffle them into new arrangements—a process known as recombination. “It’s a universal thing,” Smith said. “Every living thing has recombination systems. But at the time, no one was sure how it worked, mechanically.”
Smith chose to study recombination in a species of bacteria called Haemophilus influenza. Like many other species, H. influenzae can take up foreign DNA, either sucking in loose fragments from the environment or gaining them from microbial donors. Somehow, the bacterium can then integrate these fragments into its own DNA.
Bacteria gain useful genes in this way, endowing them with new traits such as resistance to antibiotics. But recombination also has a dark side for H. influenzae. Invading viruses can hijack the recombination machinery in bacteria. They then insert their own genes into their host’s DNA, so that the microbes make new copies of the virus.
To understand recombination, Smith produced radioactive viruses by introducing viruses into bacteria that had been fed radioactive phosphorus. New viruses produced inside the bacteria ended up with radioactive phosphorus in their DNA. Smith and his colleagues could then unleash these radioactive viruses on other bacteria. The scientists expected that during the infection, the bacteria’s genes would become radioactive as the viruses inserted their genetic material into their host’s DNA.
At least that was they thought would happen. When Smith’s graduate student Kent Wilcox infected bacteria with the radioactive viruses, the radioactivity never ended up in the bacteria’s own genome.
Trying to make sense of the failure, Wilcox suggested to Smith that the bacteria were destroying the viral DNA. He based his suggestion on a hypothesis proposed a few years earlier by Werner Arber, a microbiologist at the University of Geneva. Arber speculated that enzymes could restrict the growth of viruses by chopping up their DNA, and dubbed these hypothetical molecules “restriction enzymes.”
Arber recognized that if restriction enzymes went on an unchecked rampage, they could kill the bacteria themselves by chopping up their own DNA. He speculated that bacteria were shielding their own DNA from assault, and thus avoiding suicide, by covering their genes with carbon and hydrogen atoms—a process known as methylation. The restriction enzymes couldn’t attack methylated DNA, Arber proposed, but it could attack the unprotected DNA of invading viruses.
Even today, researchers make regular use of restriction enzymes to slice open genes.
The week before Wilcox had carried out his baffling experiment, Smith had assigned his lab a provocative new paper supporting Arber’s hypothesis. Matthew Meselson and Robert Yuan at Harvard University reported in the paper how they had discovered a protein in E. coli that cut up foreign DNA—in other words, an actual restriction enzyme. With that paper fresh in his mind, Wilcox suggested to Smith that they had just stumbled across another restriction enzyme in Haemophilus influenzae.
Smith tested the idea with an elegant experiment. He poured viral DNA into a test tube, and DNA from H. influenza into another. To each of these tubes, he then added a soup of proteins from the bacteria. If indeed the bacteria made restriction enzymes, the enzymes in the soup would chop up the viral DNA into small pieces.
Scientists were decades away from inventing the powerful sequencers that are used today to analyze DNA. But Smith came up with a simple way to investigate the DNA in his test tubes. A solution containing large pieces of DNA is more viscous—more syrupy, in effect—than one with small pieces. So Smith measured the solution in his two test tubes with a device called a viscometer. As he had predicted, the virus DNA quickly became far less viscous. Something—some H. influenzae protein, presumably—was cutting the virus DNA into little pieces.
“So I immediately knew this had to be a restriction enzyme,” Smith said. “It was a wonderful result—five minutes, and you know you have a discovery.”
That instant gratification was followed by months of tedium, as Smith and his colleagues sorted through the proteins in their cell extracts until at last they identified a restriction enzyme. They also discovered a methylation enzyme that protected H. influenzae’s own DNA from destruction by shielding it with carbon and hydrogen.
Once Smith and his colleagues published the remarkable details of their restriction enzymes, other scientists began to investigate them as well. They didn’t just study the enzymes, though—they began employing them as a tool. In 1972, Paul Berg, a biologist at Stanford University, used restriction enzymes to make cuts in the DNA of SV40 viruses, and then used other enzymes to attach the DNA from another virus to those loose ends. Berg thus created a single piece of DNA made up of genetic material from two species.
A pack of scientists followed Berg’s lead. They realized that they could use restriction enzymes to insert genes from many different species into bacteria, which could then churn out proteins from those genes. In effect, bacteria could be transformed into biological factories.
In 1978, Hamilton Smith got a call from Stockholm. He learned that he was sharing that year’s Nobel Prize in Medicine with Werner Arber and Daniel Nathans, another Johns Hopkins scientist who had followed up on Smith’s enzyme research with experiments of his own. Smith was as flummoxed as he was delighted.
“They caught me off-guard,” Smith said. “I always looked up to the Nobelists as being incredibly smart people who had accomplished some world-shaking thing. It just didn’t seem like I was in that league.”
But already the full impact of his work was starting to become clear. Companies sprouted up that were dedicated to using restriction enzymes to modify DNA. The first commercial application of this technology came from Genentech, a company founded in 1976. Genentech scientists used restriction enzymes to create a strain of E. coli that carried the gene for human insulin. Previously, people with diabetes could only purchase insulin extracted from the pancreases of cows and pigs. Genentech sold insulin produced by swarms of bacteria reared in giant metal drums.
Over the years, scientists have built on Smith’s initial successes by finding new tools for manipulating DNA. Yet even today, researchers make regular use of restriction enzymes to slice open genes. “They’re still absolutely crucial,” said Carlson. “If you want to put a specific sequence of DNA in another sequence, it’s still most often restriction enzymes that you use to do that.”
And as Smith has watched restriction enzymes become powerful and versatile, he has slowly overcome his case of Nobel imposter syndrome. “It probably was okay to get it,” he admitted.
Carl Zimmer is a columnist for The New York Times and the author of 13 books. He is currently working on a book about heredity.
This story was commissioned by the Science Philanthropy Alliance as part of its Science to Society series, illustrating the importance of basic scientific research.