I once knew a scientist who worked in the lab all of her waking hours for weeks on end. Indeed I’ve known a few. When a big discovery appears within reach, research can become an obsession. Imagine, then, what it must feel like to lose the race to be first—to be scooped. Months or years of work can become redundant, or worthless. Of no use to anyone.
While I’ve never been scooped on a big discovery in my 25 years as a working scientist, I’m fascinated by the idea of it. Because being scooped drives a wedge between two forces that motivate scientists: curiosity and ego. You get the thrill of learning the answer to the question that has obsessed you, but at the same moment you’re crushed to learn that you won’t be the one who discovered it.
One famous case, especially, captures my imagination. Not only was it one of the biggest scoops in the history of science, but the scientists involved left behind evidence of their reactions. I’m talking about the 1961 deciphering of DNA’s language, the genetic code. Sydney Brenner and Francis Crick, now famous as two of the 20th century’s most brilliant geneticists, worked on this problem from 1953 to 1961, only to be beaten to the punch by a little-known American biochemist.
In 2010, archivists released Brenner’s letters and lab notebooks, and discovered some of Crick’s lost papers mixed in. In the papers, and in the scientists’ actions, I swore I could see their reaction to being beaten to an epochal discovery: They were thrilled.
Or at least they appeared to be. I went to see Brenner to ask him.
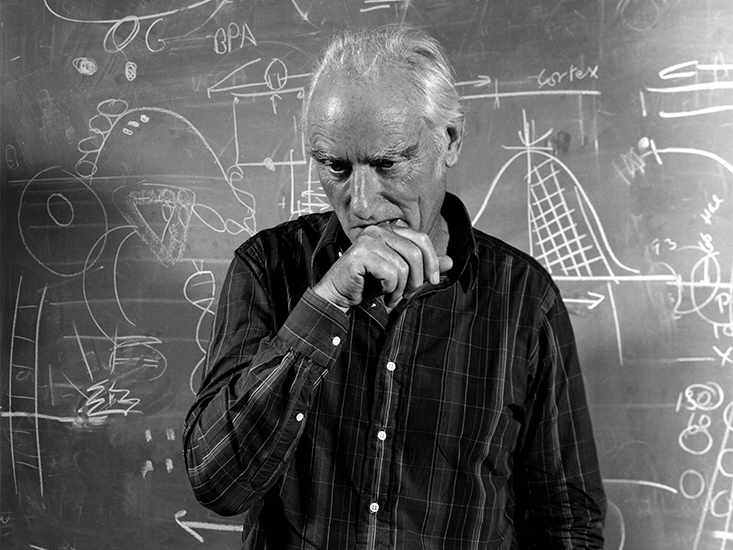
It wasn’t easy to find Sydney Brenner. He has labs and offices in Singapore, Okinawa, Virginia, and California. And he travels constantly. I found an administrative assistant near San Diego who hadn’t seen him in two years, but she knew someone. When I finally made contact, I was in luck: We’d both be in England for a day just a week and a half later.
“Come in!” he said, and I stooped into the low doorway of his home. At 88 years old, Brenner is one of the last living founders of molecular biology. He has the bushy eyebrows and knowing grin of a Santa Claus. But he can be alarmingly candid about prominent scientists whom he considers to be fools. About one, he said “I had to explain to him—listen, I thought, this moron doesn’t understand.” He has been physically slowed by age, but he’s mentally agile. I was glad I’d re-read many of his research articles from the 1950s and 60s so I could try to keep up with him.
We sat in a small, bright room crowded with plants while his stepson, Jonathan, cooked us lunch. As one of the founders of molecular biology, Brenner probably could have made himself rich if he had wanted to. But he’s well known to speak in animated tones about science, not money, and his house was modest. I felt oversized eating at the table in his tiny kitchen, where plates and mugs lined open shelves and I could reach the refrigerator from my seat.
Brenner, tapping his foot, began to take me back to how the coding problem first hooked him. A typical protein is made of a few hundred amino acids, each linked to the next like beads on a string. There are 20 different kinds of these beads. Some beads stick to certain other beads, and some stick to water, so that each string will naturally fold into a definite shape in water, forming one of the machine parts of life. There are proteins that look and work like tiny rods and levers, interlocking bricks, and wheels.
Before the early 1950s, Brenner told me, many scientists “thought that proteins were sort of fuzzy polymers,” just patterns of beads that repeated or had undefined sequences. But by 1953, proteins were found to have defined sequences of beads, and DNA looked like a secret code built from four letters: A, T, G, and C. Each gene in DNA seemed to be an instruction set for assembling a protein’s beads in the right order. The big question was, how?
The four letters of the DNA code clearly couldn’t stand for just one amino acid each, because together they could then encode no more than four of the 20 kinds of amino acids. Using two letters in succession instead—AT, AG, AC, TA, and so on—gave just 16 possible combinations, also too few. Three letters gave 64 combinations, more than needed to make 20 amino acids. So three letters was likely to be a minimum word size in the DNA, but four or more letters was possible too.
Take a look at how hard it would be to solve the code for a protein, insulin in this case, even if you knew what the DNA and the protein sequences looked like. Here’s a fragment of insulin’s protein sequence:

Today, we know that insulin’s DNA sequence looks like this:
TTCGTCAACCAGCATCTGTGTGGCTCCCACCTGGTGGAGGCGCTGTACCTGGTGTGCGGAGAGCG …
Even with this knowledge, it’s a huge challenge to decode how to get from the DNA to the protein. And in 1953, nobody knew this DNA sequence—nor any DNA sequence for that matter.
That didn’t stop people from trying to crack the code. The theoretical physicist George Gamow suggested that each amino acid bead might fit tightly into diamond-shaped holes along the middle of the DNA double helix. DNA, he thought, could serve as a physical template for stringing beads in the right order, like a row of peg holes for different shaped pegs. The dimensions were about right, and it happens that DNA’s four letters define exactly 20 kinds of these holes.
But neither Brenner nor Crick thought Gamow’s code could work, “because first of all he was reading things both ways,” said Brenner. DNA has a direction, something Gamow ignored or didn’t understand. By September 1956 Brenner discovered that Gamow’s solution couldn’t be correct, by showing that it would prevent certain amino acids from being neighbors.
Although Gamow was wrong, his idea stirred Brenner and Crick’s imaginations. Gamow thought like a cryptographer, in terms of information and codes, which was new to most life scientists at the time. The hundreds of biochemists who studied how proteins were made “were only interested in where the energy of the peptide bond comes from,” Brenner told me. Crick and Brenner, spurred by Gamow, started instead to think in terms of information transfer.
This led to some clever ideas. In February 1957, Crick suggested how the machinery inside us that reads DNA might know where each word in a DNA sequence stops and the next one starts. Even without spaces or punctuation, an English sentence made of 3-letter words like “Theboyatepieandham” is decipherable to us. That’s not just because it contains words that make sense, but also because we find only nonsense words if we start in the wrong place and count off three letters at a time. Crick proposed that in the DNA code, there might exist special “sense” words, which could dictate a reading frame to the protein-making machinery.
Crick reasoned that some combinations of letters couldn’t possibly serve as sense words. For example, an amino acid specified by AAA wouldn’t work because two such words in a row, AAAAAA, would make the reading frame impossible to find. To build a set of possible three-letter words from just A, T, G, and C, a colleague of Crick’s calculated that not just AAA but 43 more of the 64 possible three-letter combinations would need to be excluded as nonsense words—leaving exactly 20 possible three-letter words, the magic number of amino acids. It was a promising and clever solution, but it turned out to be wrong. We know now that almost all words are sense words, including words made of repeating letters. Proposals like this one, though, were instrumental in shaping clear questions about the code.
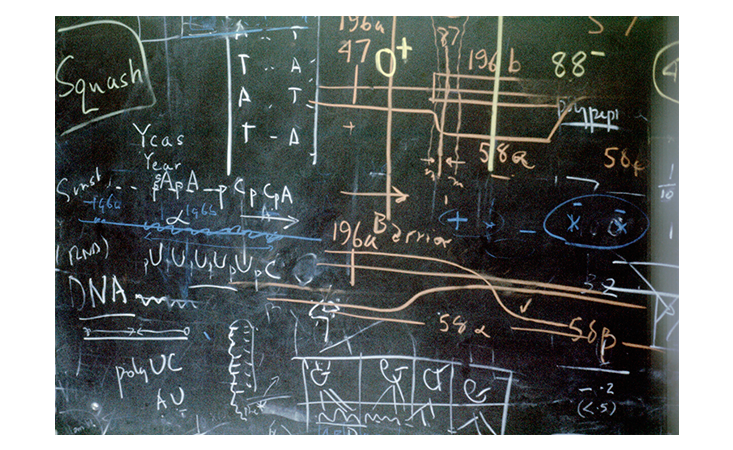
In 1958, Brenner thought of a way to move from theoretical proposals to tinkering directly with DNA. Thinking about a colleague’s results on DNA viruses, he made a prescient guess that a bright yellow dye called proflavin might produce single-letter deletions or additions in DNA, and that these might knock the reading of DNA out of frame. If the reading frame mattered, then the protein produced would make no sense—think “The boy ate pie and ham” becoming “Heb oya tep iea ndh am.” He and Crick started working together in the lab, growing DNA viruses on petri plates. The viruses would infect bacteria, leaving a characteristically sized hole on a lawn of bacteria when the protein in the DNA virus was working properly—if the change in the DNA hadn’t disabled the resulting protein. “That you can predict from these holes things about the internal structure of DNA,” Brenner told me, “has always seemed to me to be remarkable.”
Brenner and Crick combined mutants and found that most of the dye-induced mutants could be suppressed by certain of the other dye-induced mutants. Reasoning that the dye had sometimes added a letter and sometimes deleted a letter, they started calling the changes “+” and “-”, which they had to assign randomly since knowing which was which was impossible. Combining + with – often reverted the viruses back to normal, as if this restored a normal reading frame by gaining and losing a letter in the same stretch of sequence, whereas combining + with + or – with – never did.
The results were making sense. If their theory was right, then Brenner and Crick were on track toward finally breaking the code. They couldn’t read individual letters in the DNA, but after eight years of work, it looked as if they could at least change letters in predictable ways. Brenner worked night and day. Crick, who rarely did experiments, became consumed by them too, on some days walking back from the lab through Cambridge to visit his home only for meals and to sleep.
Then the surprise came.
In August 1961, more than 5,000 scientists came to Moscow for five days of research talks at the International Congress of Biochemistry. A couple of days in, Matt Meselson, a friend of Crick’s, told him the news: The first word of the genetic code had been solved, by somebody else. In a small Friday afternoon talk at the Congress, in a mostly empty room, Marshall Nirenberg—an American biochemist and a complete unknown to Crick and Brenner—reported that he had fed a single repeated letter into a system for making proteins, and had produced a protein made of repeating units of just one of the amino acids. The first word of the code was solved. And it was clear that Nirenberg’s approach would soon solve the entire code.
Here’s where I like to imagine what I would have done if I were Crick. For someone driven solely by curiosity, Nirenberg’s result was terrific news: The long-sought answer was arriving. The genetic code would be cracked. But for someone with the human urge to attach one’s name to discoveries, the news could not have been worse. Much of nearly a decade’s worth of Crick and Brenner’s work on the coding problem was about to be made redundant.
I’d like to believe I would have reacted honorably. I wouldn’t have explained away Nirenberg’s finding to myself, concocting reasons why it wasn’t convincing. I wouldn’t have returned to my lab and worked a little faster to publish my own work sooner. I’ve seen scientists react like this to competition. I’d like to believe that I would have conceded defeat and congratulated Nirenberg. Of course, I’ll never know what I would have done.
Crick’s response was, to me, remarkable and exemplary. He implored Nirenberg to give his talk again, this time to announce the result to more than 1,000 people in a large symposium that Crick was chairing. Crick’s Moscow meeting booklet survives as an artifact of his decision, with a hand-written “Nirenberg” in blue ink, and a long arrow inserting into an already-packed schedule the scientist who had just scooped him. And when Nirenberg reached the stage, he reported that his lab had just solved a second word of the code.
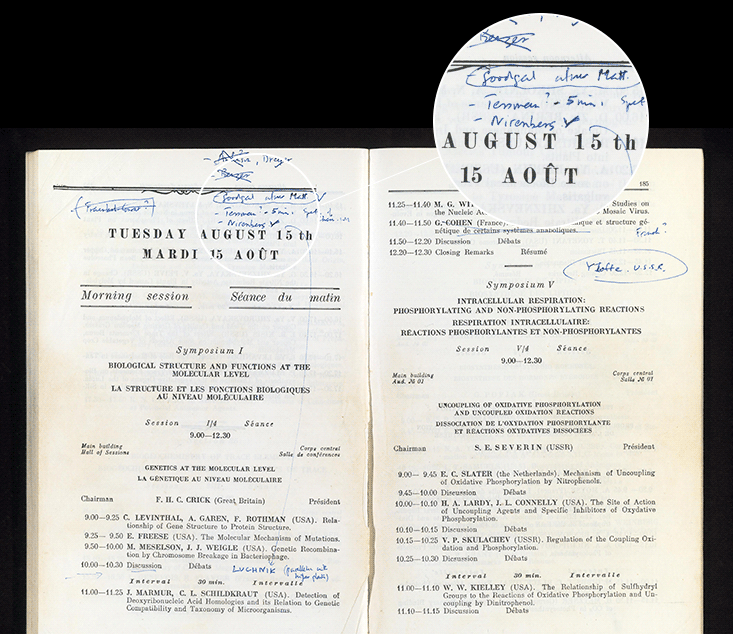
Crick would later write that the audience in Moscow had been “electrified” by Nirenberg’s result, changing the word later to “startled.” Crick may have felt both startled and electrified. But disappointment? If Crick felt some disappointment, it wasn’t apparent to Meselson, who characterized Crick’s initial reaction to the news as “joy.” “What else could it be?,” Meselson explained to me by phone, “by god, we’d know the answer in our lifetime!”
Even Meselson, who had his own research program on DNA, had impulsively hugged Nirenberg at the end of his talk. I tried to imagine two men of that era spontaneously hugging in a lecture room at a conference, particularly when one was a geneticist and other a biochemist. Maxine Singer, a biochemist who gave Nirenberg the RNAs he needed for his experiments, told me that the “biochemists and geneticists were suspicious of each other,” something that Brenner echoed to me in person.
Soon after the Moscow meeting, Crick and Brenner made an important contribution. They knew by then that inserting or deleting one or two letters in viral DNA would disable the resulting protein. But now they saw that inserting or deleting three letters could make it work again, as if this restored the correct reading frame. The result implied that the DNA code was composed of words three letters long. This was an important component to solving the code, perhaps as important as Nirenberg’s discovery.
Letters from Crick to Nirenberg from 1962 reveal that Crick and coworkers did try doing experiments like Nirenberg’s for a short time, which made me wonder if he was trying to stake out some credit for discovering the code. But these letters and Crick’s text for a 1962 BBC radio address both made clear what he felt—it was Nirenberg’s group who deserved the credit for making the real breakthrough. “No, he definitely believed it,” Brenner told me, when I asked if Crick was just being kind. I asked Brenner what his own reaction was to hearing Nirenberg’s result. “We thought this was very significant … It was very good,” he said. I thought he was being stoic, but as I pressed him again and we talked further, I became convinced that he, too, had really had been thrilled to learn the answer.
It’s hard to overstate the importance of the decoding work done by Nirenberg and his colleagues. Most of the major advances in modern biology that have happened since rely on it. My own lab uses it every day. It is to molecular biologists what the periodic table is to chemists—the foundation for all future discoveries. Of course Nirenberg built on the work of others before him, and many other scientists, including Brenner and Crick, refined his result with other important discoveries. But here was landmark science. And the people who were beaten to the answer were excited. Because “then we could get on with more important problems,” explained Brenner.
It’s at least as easy to lose a result today as it was in 1961. We try not to do research that’s redundant. But multiple scientists are often motivated by the same questions, so we sometimes can find ourselves racing each other. And losing a race has consequences. Funding can be endangered. Careers can be derailed. We can feel the tension, on occasion, between what’s good for the individual scientist and what’s good for the progress of science. Crick and Brenner’s reaction stands as an examplar for me, as it should for all scientists. As Brenner told me by way of parting advice: “Do the best experiments you can, and always tell the truth. That’s all.”
Bob Goldstein heads a biology research lab at the University of North Carolina at Chapel Hill.