Of the important global crops, the banana is the most genetically uniform. A single cluster of nearly identical genotypes, the Cavendish subgroup, nearly monopolizes the world’s banana groves and banana trade. In contrast to the riotous rainbow of genetic diversity that lends sustainability to natural plant and animal populations, the world’s banana industry has the stability of an upside-down Egyptian pyramid balanced on its tip.
That fact leads to another superlative: The commercial banana is the world’s most endangered major crop. The future of the intercontinentally traded banana was once, and is again, precarious. Given that their wild progenitors are as variable as most species, how has it come to pass that most of the banana plants growing in the world have become so uniform? And what does that uniformity mean for their future as the “world’s most perfect food”?
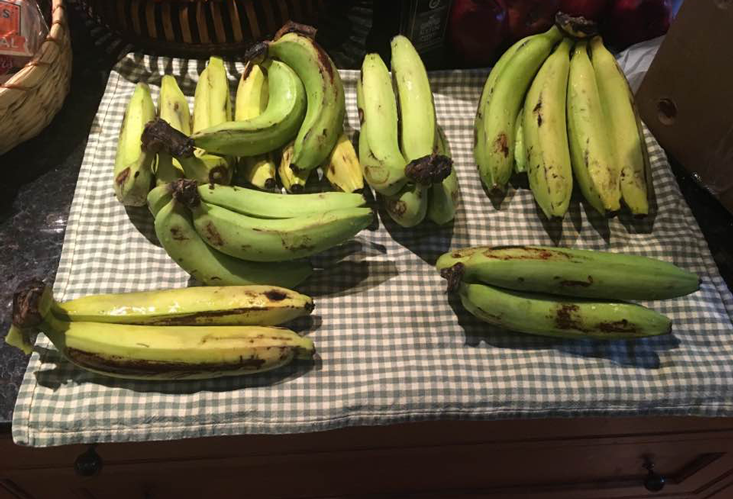
Reproductively, domesticated banana plants are self-copying machines. Proto-farmers who found an individual whose fruit they liked (maybe tasty and not so seedy) could dig up its suckers and replant them in a nearby clearing or even along the trail. In a few years, an industrious proto-farmer might have had reproduced dozens of plants of that genet.
Skip ahead a few thousand years. During the Age of Discovery and Conquest, that model took on global proportions as plant explorers transported a relatively small number of favorite clones out of Southeast Asia and introduced them to the plantation agriculture of tropical colonies. With the emergence of the 20th century, the confluence of the Industrial Revolution with plantation agriculture led to the propagation of a single globally favored banana genet (descended from a single instance of sperm-egg fusion) for export from the tropics to waiting markets in the industrial north. Foreshadowing the uniform and reliable vehicle of Henry Ford, the banana industry had found the uniform and reliable banana clone. Everyone was eating the Gros Michel banana (aka Big Mike).
If you ask any long-term restaurateur, you will find that the secret to getting customers to come back again and again depends on making their favorite meal exactly the same again and again.1 Constant change—for example, a port-cherry sauce on the duck this week and a lime-dill sauce the next—will send patrons scurrying. If the newspaper food critic’s blog tells you to try the tempeh, you won’t be pleased to hear that it was replaced by tofu. Part of fast food’s global success is that you pretty much know what you are getting. A McDonald’s burger and fries meal hardly varies from Riverside, California, to Elk Grove, Illinois, to Uppsala, Sweden. (Actually, McDonald’s fries in Sweden are a pleasant surprise.) We are especially dismayed by nasty shocks such as the apricot that never ripens properly or the clementine mandarin with a dozen seeds.
Some plant biotechnologists have argued that future crops should follow the banana, dispensing with sex entirely.
From farmer to grocer, the whole modern industrial food production and delivery stream depends on predictable uniformity. For field crops, seeds must germinate at the same time, and plants must grow uniformly. Grain must mature uniformly so that a farmhand swinging a scythe or driving a combine can harvest a field in a single sweep. For horticultural crops, packing lines work best when fruits have predictable shapes and sizes to be sorted into standardized boxes. Retailers don’t like it when some fruits arrive ripe, others are immature, and the rest are rotten. Given a choice, most customers will leave the oddly shaped tomato or the unevenly colored orange in the bin.
The major sources of biological variation are environmental differences and genetic differences. Industrial farmers have become increasingly sophisticated in dealing with environmental variation. The cutting edge known as precision agriculture enables a farmer to treat every plant as uniformly as possible. Combining precise spatial information from a Global Positioning System (GPS) with high-tech field machinery can be used to alter the external environment (flatten that field). Add soil and plant sensors for nutrient and water status, mix with Internet cloud-based data analytic tools, and you’ve got a recipe for undoing some of Mother Nature’s environmental tweaks.
With these modern tools, crop, land, and irrigation management can attain a refined level of environmental uniformity. But there’s a limit to controlling for uniformity if a crop is genetically diverse and that diversity is manifest over plants and their products. Genetically variable outcrossed sexual seeds throw off a tremendous amount of variation. We enjoy the kaleidoscope of colored kernels on an ear of dried “Indian corn” for a Thanksgiving decoration. But a kaleidoscope of interplant variation is a nightmare for those seeking to make their product as predictable and uniform as possible. However, not just any single genet will do. Some genotypes are developmentally unstable. They simply won’t be uniform, no matter how nicely you treat them. Others might be uniform within any given locality, but vary among localities—sensitive to what might seem minor variations in terroir. From the point of view of a global banana industry, the very same banana (i.e., in the eyes of customers as well as those who pack, move, and sell the product) must be produced in Ecuador, Philippines, Guatemala, Costa Rica, and Colombia.
For the commercial banana industry and trade, the Gros Michel genet must have seemed like Mary Poppins, “practically perfect in every way.” It was a tasty tropical treat for 19th-century urban customers who enjoyed their fresh fruit in the summer but had to turn to canned or dried fruit in the winter. With a thick skin, it traveled well. More importantly, the variety grew and yielded well and uniformly in a variety of tropical countries and continents. After harvest, it survived the long boat ride to cooler climes, delivering a good banana for the first half of the 20th century. Peter Chapman explains:
United Fruit [Company] was a pioneer of mass production. With its one-size-fits-all banana, the company beat Henry Ford, the man often credited as the pioneer of standardization, by a number of years. Big Mike was on the shelves at the turn of the 20th century; the Model T, on the other hand, rolled off the production line in 1908. … United Fruit’s bananas were the forerunners of those products we know today: the cup of cross-cultural coffee foam; the multi-national hamburger.2
Because most important crops reproduce only by sexual seed, they cannot be clonally propagated. Not surprisingly, the genetic variation generated by sexual reproduction is an obstacle for many folks looking to deliver a better crop product. For the past quarter century, some plant biotechnologists have argued that future crops should follow the banana, dispensing with sex entirely. Specifically, they are titillated by the idea of varieties that replicate the maternal plant via reliably uniform, asexual, apomictic seed.3 One proposal is that the plant breeders would maintain sexually fertile lineages that, when crossed, would create apomictic offspring. A second approach would be to genetically engineer plants to be apomictic. The seeds produced by either method could be delivered to farmers, who benefit from the crop’s uniformity.
The explanation for sex isn’t straightforward. Sex is a hassle.
Depending on whose prose you read, asexual seed could be a real boon to farmers—or not.4,5 Some scientists see social justice in apomictic seed.6 Presently, the vast majority of seed of outcrossed crops are so-called hybrid varieties. Hybrid varieties are high producing and highly uniform, resulting from crosses between two highly uniform inbred varieties. (Note: Commercial hybrid varieties don’t have anything to do with hybrids between species.) But when these plants from hybrid seed mate with each other, sex scrambles their genetic structure apart. The offspring are both variable and inferior. Decades ago, I visited a Mennonite farm near Lancaster, Pennsylvania, and saw struggling dwarf corn plants on the edge of a productive field. The farmer explained that these dwarfs were the sexually mixed-up seed that had fallen into the soil from the prior year’s hybrid crop. Despite receiving the same rainfall, weed removal, and fertilizer, the volunteer plants were less than half the size of the hybrid crop plants growing just inches away.
Farmers can’t get a decent crop by saving and replanting the seeds produced from a hybrid variety. If they like what they have and can afford it, farm operators must return to buy more from the seed company every year. Farmers in poverty are at a disadvantage. If they can ever afford to buy hybrid seeds once, their onetime gains would still be insufficient to help them to buy new seeds every year. With apomictic varieties, farmers wouldn’t have to buy seeds year after year because their hybrid crops would produce progeny identical to and as vigorous as their mother. Proponents for this scenario are obviously in the public research domain—for example, nonprofits and governments. A prominent example is the independent, nonprofit Cambia, an institute that is exploring how apomictic varieties can be created by genetic engineering and more advanced molecular technologies.
But given the cost and difficulty of engineering apomixis, there is the concern that corporations that could bear the cost might create and use apomixis technology for their own profit-oriented purposes, and that poor farmers might not be able to access the expensive products. There is also the question of whether asexual seed is always a boon. A farmer who buys a sexually sterile apomictic variety has no opportunity to engage in his own crop improvement. That’s especially true for farmers in developing countries who don’t routinely use hybrid varieties. Instead they grow open-pollinated landraces that evolved constant experimentation. The most experimental farmers grow their crops as a mixture of plants descended from saved, exchanged, and even purchased seed, often actively or passively participating in activities that increasingly adapt their crops to local conditions. Concern about corporate control of apomixis led to the 1998 “Bellagio Apomixis Declaration,” urging “widespread adoption of the principle of broad and equitable access to plant biotechnologies, especially apomixis technology,” and encouraging “the development of novel approaches for technology generation, patenting, and licensing that can achieve this goal.”7 At the moment, plants genetically engineered to create apomictic seeds are lab-bound, a long way from the field and even a longer way from distribution to farmers.
One thing that everyone agrees on is that a clonal crop is genetically very uniform. That’s both the good news and the bad news because, despite its tremendous popularity, the Gros Michel is nearly gone. Not because a better-tasting or a better-yielding banana came along but because, according to banana geneticist and crop evolutionist N. W. Simmonds, “bananas constitute one of the best examples in the history of agriculture of the pathological perils of monoclone culture.”8
Life without sex can have its downsides.
“What use is sex?” “What good is sex?” “Why sex?” “Why have sex?” Are these complaints of a frustrated celibate? No, they are titles of scholarly academic papers dating from the last half of the 20th century and continuing to the present day. While sex may be a bit of a mystery to us all, it has been the grand enigma of evolutionary biology for decades.
Sex is nearly ubiquitous, but it hasn’t been easy to explain its ubiquity.
It is a habit of evolutionary biologists to explain the persistent and widespread properties of organisms with a bit of Darwinian logic. Prominent characteristics are readily explainable as adaptations. You find plants that conserve water in the desert. Animals with spots survive and flourish in habitats dappled with light and shadow. Light-skinned folk originate from cloudy areas. When they live in sunny climes, they suffer sunburn, skin cancer, and vitamin D poisoning. Darker-skinned folk who tend to be native to regions with intense sunshine are far less vulnerable to these problems. Sexual reproduction is a common feature of all major groups of organisms because … um … because … well … hmmm?
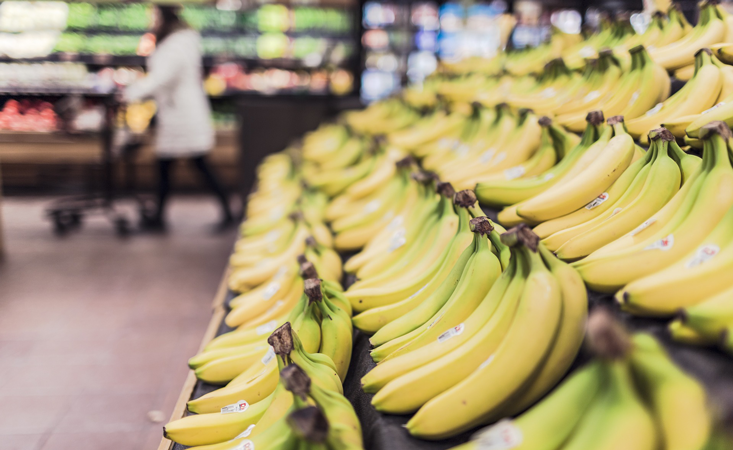
The explanation for sex isn’t straightforward. Sex is a hassle. To reproduce without sex, an organism can dedicate a cell toward creating a new individual, pump it up with some resources, and eventually set its baby free. The organism that uses sex to reproduce has a greater challenge; it has to create gametes that have to find other gametes. The process of seeking or attracting those other gametes typically involves allocation of resources to special structures and, in the case of animals, allocation of resources and time to special behaviors.
To evolutionary biologists, sexuality involves an additional hassle. Sex appears to violate the rule that an individual bearing a Darwinian adaptation cannot be successful unless it leaves more genes than the alternate trait. (According to Darwin, “for the good of the species” is not good enough.) For sexual reproduction to serve the evolutionary benefit of an individual, organisms engaging in sex should leave more of their genes to the next generation than those that reproduce without sex.9 The alternate trait is asexuality, and that should leave more genes than sex.
The paradox is explained by simple arithmetic. An individual that creates a seed by apomixis passes on all, 100 percent, of its genes. An individual that creates a sexual egg puts half, 50 percent, of its genes in that basket. The fertilizing sperm from another individual makes up the other 50 percent. The sexual seed passes on half as many of its maternal genes than does an asexual seed (or any other asexually produced offspring). Evolutionary biologists did their arithmetic and gasped. An individual that procreates asexually passes on twice as many of its genes per offspring as the one that procreates sexually. Dispensing with sex yields an immediate twofold benefit. A similar, but more complicated, mathematical argument comes to the same conclusion for organisms that have the option of self-fertilization. For sexual reproduction to be maintained in a species, its benefit must be huge and obvious. A myriad of theories have been developed—and continue to be developed—suggesting what that “obvious” benefit might be. Most proceed from the simple fact that sexually produced offspring are genetically different from each other and from their parents and ask the question “What’s the advantage to being different?” The theories can be crudely lumped into three groups.
One approach, nicknamed the Lottery model, says that the asexual parent is analogous to an individual who buys a pile of lottery tickets, but each with the same number. The sexual parent takes the alternative strategy of buying a pile of tickets that are all different from each other. If the winning conditions are very predictable, that is, the environment for the progeny is identical to that of the parent; the asexual parent is the big winner. But if environments change unpredictably in time and space, the sexual parent has a better chance of holding the winning ticket. Notice that this argument works well for those individuals who have lots of progeny (tickets) and those progeny end up in unpredictable environments. It works especially well if progeny end up dispersed away from the maternal environment.
The next model requires that siblings interact and compete over some set of limited resources. The Elbow Room model proceeds as follows: If all the progeny are identical, they all have the same needs and specialized adaptations. In those organisms that have limited dispersal, asexual progeny are likely to hurt each other by equally depleting the same critical resources. In contrast, sexually produced progeny have somewhat different resource adaptations and needs from each other and will probably interfere less with each other as much as those that are identical. Three mechanically endowed sisters in a small and remote west Nebraska town are less likely to prosper setting up competing Ferrari repair shops compared to three siblings diversely talented as butcher, baker, and candlestick maker.
The only other solution was to either find or build a better banana.
The final model starts with the premise that the world is full of biological enemies: predators, parasites, and disease organisms. But biological enemies do not obtain their nutrition willy-nilly. These organisms have specializations for finding and attacking their prey and hosts. Under these circumstances, it is good to be different from others of your species and, if dispersal is low, from your siblings. It’s good to be the rare type in the “eyes” of the enemy, that is, inconspicuous. Let’s consider a crude example that involves a predator. A bird that is visually attuned for attacking bright blue beetles might miss those few that are dull green, brown, et cetera. An asexual bright blue beetle and its kids are doomed, but a sexual blue beetle has the opportunity for sex to shuffle the genes with the evolution of novelty every generation. Under strong evolutionary pressure from a biological enemy, the rare type becomes the common type. Sex might be the trick to provide temporary escape for some progeny in the next generation, as natural selection begins to favor the birds that are visually attuned for dull colors.
Disease organisms—worms, bacteria, fungi, and other simple organisms—not predators, are thought to run the show. They have short generation times (as rapid as once every 20 minutes) and thus can come in huge numbers (bazillions or more). While most have the option of sex, many get their variation from high rates of mutation (spontaneous genetic change). That combination allows for blazingly fast evolution by natural selection. They evolve to pursue and mow down the most common susceptible host genotype (or set of similar genotypes). The once-rare host type becomes common. Then selection sorts among the variation in the now-beleaguered disease organism. The once-rare-but-now-common host gets hit with the newly evolved disease organism. And so the evolutionary cycle continues.
A sexually evolving host cannot rest (and certainly cannot revert to asexuality) when pursued by rapidly evolving disease-causing microbes. In this co-evolutionary race, both the disease organism and host are running as fast as they can to stay in one place. And that’s why the theory is referred to as the Red Queen model, after a broader co-evolutionary theory (the Red Queen hypothesis). The theory’s name pays homage to the Red Queen’s race of the same type in Lewis Carroll’s Through the Looking-Glass, when she says:
“Now, here, you see, it takes all the running you can do, to keep in the same place. If you want to get somewhere else, you must run at least twice as fast as that!”
A massive body of experimental and descriptive scrutiny of these major models, their variants, and some other hypothesis has accumulated over the last handful of decades. Lively and Morran have thoughtfully reviewed the state of the art.10 While the definitive answer may not yet have arrived, a consensus is certainly emerging. The Elbow Room model appears to be the big loser. Several experimental studies have been conducted involving competition between sets of asexual and sexual progeny from the same mothers. They rarely show an advantage to the genetically more diverse sexual offspring; at best, whether increasing genetic diversity reduces competition appears to be idiosyncratic and hardly universal. The Lottery model appears to have some validity in the minority of cases examined, especially for those organisms that create vast numbers of progeny that are widely dispersed. With tremendous support from studies of natural populations and strong support from experimental studies, the Red Queen is the big winner. Data from both plants and animals reveal that there’s an advantage to being the rare genotype in the presence of a biological enemy. Many of the studies that actually measured the evolutionary benefit, in terms of number of genes represented in the next population, have shown that sex’s advantage—in the face of biological enemies—often exceeds the twofold evolutionary cost of sex.
It turns out that the Red Queen has something to say about bananas.
Lest we forget: “Bananas constitute one of the best examples in the history of agriculture of the pathological perils of monoclone culture.”8 The Red Queen is behind the demise of the Gros Michel. Once you have huge swaths of extreme biological uniformity, it is only a matter of time before some pest stumbles upon that sea of free reliable and uniform nourishment. In the case of the Gros Michel clone, the culprits were two fungal species—one that caused Panama disease (even though it was first recorded in 1876 in Australia) and another that caused a disease called yellow Sigatoka. It turned out that the billions of Gros Michel plants were fully susceptible to both of these as well as an array of minor pests. The Panama disease organism was—and continues to be—particularly nasty. No control methods have yet been found to save infected susceptible banana genotypes. Panama disease destroyed most of the Gros Michel plantations in the Americas in the first half of the 20th century.11 Faced with rapidly disappearing bananas, the only other solution was to either find or build a better banana. The quest began in earnest in the 1920s. That was the same time that “Yes! We have no bananas” became popular. But whether the impending demise of Big Mike was the inspiration for the song remains a matter of speculation.
As the Gros Michel lurched to near-extinction, the fruits of those research programs began to be planted. Gros Michel was replaced with a set of very closely related resistant genets (all, in fact, are clonally descendants of a single genotype, all mutants, no sex involved) known as the Cavendish subgroup. Cavendish was a preexisting clone that wasn’t susceptible to the nemeses of Gros Michel. Today, the Cavendish subgroup rules. Roughly half of the world’s 9 million acres of bananas belong to this group. Furthermore, Cavendish types are the banana of intercontinental trade. Currently, cultivation of Gros Michel hangs on in parts of the world where those nasty fungi haven’t yet found it.
But the Red Queen’s race doesn’t stop. Organisms that stand still, like the billions of Cavendish plants, will eventually fall behind. As Cavendish began to rise to dominance, new disease organisms started attacking it. The worst contemporary banana disease is black Sigatoka, caused by a close relative of the yellow Sigatoka fungus.12
More worrisome in the long run is an old friend. The original Cavendish-resistant Panama disease culprit has now been named Race 1. Panama disease fungi have evolved; the Race 1 genotypes are being replaced by one known as Race 4, which first appeared in 1965. Cavendish has no resistance to Race 4. The evolutionarily new and improved Panama disease organism has wiped out thousands of Cavendish acres in Southeast Asia. Since then it has been identified in the Pacific, Australia, Africa, and the Middle East. Worse yet, in 2011 Cavendish bananas in India started succumbing to what appears to be a new genetic variant of Race 1. The bad news is that Cavendish is fully sexually sterile.
Norman C. Ellstrand is a distinguished professor of genetics at the University of California, Riverside, where he holds the Jane S. Johnson Endowed Chair in Food and Agriculture. He is the author of Dangerous Liaisons?: When Cultivated Plants Mate with Their Wild Relatives.
Reprinted with permission as an adapted excerpt from Sex on the Kitchen Table: The Romance of Plants and Your Food by Norman C. Ellstrand, published by The University of Chicago Press. © 2018 by The University of Chicago. All rights reserved.
References
1. Bourdain, A. Kitchen Confidential: Adventures in the Culinary Underbelly Bloomsbury, New York, NY (2000).
2. Chapman, P. Bananas: How the United Fruit Company Shaped the World Canongate Books, Edinburgh (2007).
3. Hand, M.L. & Koltunow, A.M.G. The genetic control of apomixis: Asexual seed formation. Genetics 19, 441-450 (2014).
4. National Research Council. Environmental effects of transgenic plants. National Academy Press, Washington, D.C. (2002).
5. National Research Council. Biological confinement of genetically engineered organisms. National Academy Press, Washington, D.C. (2004).
6. Jefferson, R.A. Apomixis: A social revolution for agriculture? Biotechnology and Development Monitor 19, 14-16 (1994).
7. Group of Reproductive Development and Apomixis. “Bellagio Declaration.” Laboratorio Nacional de Genomica Para la Bio (1998).
8. Simmonds, N.W. Evolution of Crop Plants, 2nd Edition Smartt, J. & Simmonds, N.W. (Eds.) Longmans, Harlow, U.K. (1995).
9. Williams, G.C. Sex and Evolution Princeton University Press, Princeton, NJ (1975).
10. Lively, C.M. & Morran, L.T. The ecology of sexual reproduction. Journal of Evolutionary Biology 27, 1292-1303 (2014).
11. Stover, R.H. & Simmonds, N.W. Bananas, Tropical agricultural series, 3rd ed. Longmans, London (1987).
12. Robinson, J.C. Bananas and Plantains CAB International, Cambridge (1996).