At some point in our lives, we are confronted with something we fear is larger than ourselves, and with nowhere else to turn, we peer up to the heavens. There are also those among us who look there first and hold that gaze for a lifetime.
Astrophysicist Mario Livio is one such person. A senior theoretical astrophysicist at the Space Telescope Science Institute, Livio is an expert on supernovae and their imaging. His work has helped to determine the expansion rate of the universe and to understand dark matter and black holes.
I was there at NASA’s Kennedy Space Center in 1990 when Hubble became the first major optical telescope to be launched into space. Our so-called “eye in the sky” allowed us to look back in time and illuminate our origins. In spite of a rocky start that required a repair mission, Hubble soon detected evidence of massive black holes, followed by Pluto’s moons, and then the universe’s early galaxies.
In celebration of the Hubble Telescope celebrating its 25th anniversary this year, I spoke with Livio at the Institute about the mission’s extraordinary achievements and its impact on science, the arts, and society. Livio took me on a tour through distant supernovae, dark energy, dark matter, the Hubble Constant, and galaxy evolution, demonstrating his trademark ability to make complex science understandable.
As for the most revolutionary question he sees facing mankind? Whether we are alone in the universe.
Each video question plays at the top of the screen.
View Video
How has the Hubble Space Telescope changed the way we see the universe?
Hubble’s images, M16 and Deep field, are particularly well-known. What other images are just as mesmerizing?
How does Hubble’s Cosmic Origins Spectrograph show us the structure of the universe?
Ground-based telescopes can provide different information than space-based telescopes. Can you explain?
What is dark energy?
What will dark energy do to our universe?
What is dark matter?
What has Hubble discovered about black holes?
Hubble has the ability to look very deep into the universe. What do Hubble deep fields show?
What does this ability to see so far into the universe mean for the discovery of other habitable planets?
How has the prospect of completely decommissioning Hubble affected you?
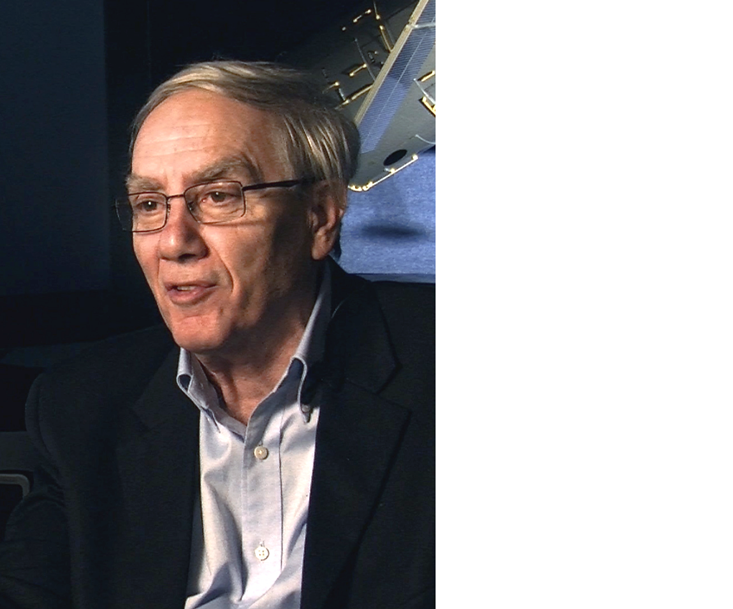
Interview Transcript
How has the Hubble Space Telescope changed the way we see the universe?
We’ve known since the 1920s, that our universe is expanding; but we thought that maybe this expansion is slowing down because of the gravity of all the mass within the universe. What we discovered in 1998—and Hubble played a very crucial role in that discovery—is that not only is the expansion not slowing down, it is actually speeding up; it’s accelerating. And this acceleration is caused by this repulsive force, as opposed to gravity’s attractive force, of this thing we call dark energy.
Now, how was that discovered? Basically, we look at very distant stellar explosions that we call Type 1a Supernovae. Here you can see some of them. Here, you see a galaxy. You see that point of light appearing there? That’s the supernova. Or, here is a galaxy. You see that point of light appearing there? That’s the supernova. Now, these are very distant supernovae. They are distances of maybe 7, 8 billion light-years away. What that means is that the light left those supernovae 8 billion years ago. So we get a picture, if you like, of our universe as it was 8 billion years ago. So we can compare the expansion that [existed] then to the expansion that is now—and that’s how we found basically, that the expansion is accelerating, speeding up.
Another very important thing that Hubble has done was to refine measurements of the Hubble constant. The Hubble constant is actually a measure of the current rate of expansion of the universe, and it is the most important parameter that determines the age of the universe. The age basically goes like one over the Hubble constant.
Before the Hubble Space Telescope was launched, there were two groups of astronomers who were quarrelling all the time about the value of the Hubble constant. They had two values that were different, one from the other, by a full factor of two. In the appropriate units, one had a value of about 50 and one had a value of about 100, which of course cannot be. What happened with the Hubble Space Telescope is [that] by lots and lots of measurements, of many, many distant indicators—like in this galaxy, these green circles, they mark the locations of things we call Cepheid Variables (these are stars that pulsate)—it turns out that there is a very, very tight [relationship] between the period of the pulsation (how long it takes the star to pulsate) and the actual brightness of the star, the intrinsic brightness of the star. Because we can measure the period of the pulsations quite accurately, we can know what the real brightness of the star is. And then, by comparing the real brightness of the star to the brightness that we see, we can tell how far away [that] star is. It’s like [this]: If I hold a candle here, and I hold it at twice the distance, it would be four times less bright. It falls like, one over the square of the distance—the brightness of the thing—and this is with these things as well.
We can then determine the distances and that has been the main problem: Determining distances with high precision. But now we have done this. Currently, we have managed to refine the value of the Hubble constant. We know it within an error of no more than about 3 percent. Remember, from knowing it only to within a factor of two, to knowing it within 3 percent. And, that’s how well we actually now know the age of the universe. The age of our universe is very nearly 13.8 billion years, and we know that now with a very, very high precision.
Hubble’s images, M16 and Deep Field, are particularly well known. What other images are just as mesmerizing?
Well, first of all, I would say that those two images are still mesmerizing, I think, yes? M16 has become iconic; there is no question about that. It has been called, “The Pillars of Creation,” and so on. I mean, these columns of gas and dust where new stars are being born—they are really fantastic. The Hubble Deep Field, and its successors, the Hubble Ultra Deep Field, eXtreme Deep Field and so on, continue to be very inspiring, showing us that our universe is [made up of] hundreds of billions of galaxies and so on, so certainly amazing. But of course, there have been many more since then. Here are a few of them.
So this one, we have called this Mystic Mountain, simply because it looks like a mountain! It’s another pillar of gas and dust where new stars are being born inside. Not only that new stars are being born inside, but here, you can even see the jets that emanate from the disc around a young star that is being born inside there. And here, you can see another jet that emanates from another star that is being born inside here, and [which] has this jet in it. And of course, since the cameras that we have installed on Hubble through servicing missions since then have a higher resolution and better image quality, then of course, the images give many more details than we were able to see before. I think this is just an astounding image, Mystic Mountain.
There are other fantastic images. Like this. This is the Horsehead Nebula, called that because in ground-based images, and so on, this looked a little bit like the head of a horse. But we have now taken this image in infrared light because Hubble has the Wide Field Camera 3, which has infrared capabilities. Dust is completely opaque to visible light, but it is mostly transparent to infrared light. What infrared allows is [the ability] to see through parts of this nebula and therefore, see all [of] these incredible details in this Horsehead. I believe this is a truly amazing image.
Another image that I think is fantastic is this. We sometimes call it, “The Rose.” These are really two interacting galaxies, and because of their gravitational interaction, because of tidal forces, each one of them stretches a bit the other and they form this structure that looks a little bit like a rose, so we call it that. Again, you can see here in all those tails, in those tidal tails, all those blue clusters of stars, new stars being born and so on. All of this happens as a result of this interaction. So we really have literally thousands of new images, and many of them are really breathtaking.
How does Hubble’s Cosmic Origins Spectrograph show us the structure of the universe?
Dark matter is really responsible for the formation of structure. What happened in the early universe is [that] it’s the dark matter that collapsed first to form certain gravitational potential wells, and the ordinary matter just then flowed into those potential wells. That’s how eventually, galaxies started forming and clusters of galaxies started forming.
But when we simulate this structure that this dark matter forms—and now there are very, very large computer simulations that do this—we discover that it forms in like a network of filaments, a cosmic web of filaments of this dark matter. It’s a bit like a sponge, but it’s really where the filaments would be the thing, and between them, there are these voids and so on. So we have this kind of structure and then the ordinary matter collects at those densest points in that cosmic web-type thing.
Now, how can we discover the existence of this cosmic web? Eventually, all the intergalactic gas forms this type of thing. We cannot see it because it doesn’t emit enough light for us to actually be able to see that. But we look at more distant quasars for example, which are these very, very distant black holes at centers of galaxies, which appear like points of light. And the light from those quasars passes—on its way to us, to our telescope—it passes through many of those filaments. As it passes through the filaments, atoms manage to absorb some of the light from that light that comes in, and we actually see these absorptions in the spectra that we take. So Hubble, for example, has this fantastic spectrograph now called COS, Cosmic Origins Spectrograph, which really is seeing all these things and thereby is allowing us to map this three-dimensional structure of the cosmic web that we have.
Ground-based telescopes can provide different information than space-based telescopes. Can you explain?
Each one of those telescopes has its strengths and its weaknesses. The telescopes on the ground are very large. The Keck telescope, the Very Large Telescope—they have large collecting areas and therefore, they can go after very faint things. If you need to take a spectrum of a very faint object, that’s the way you’re going to do [it]. On the other hand, in visible light … Even though adaptive optics has managed to take out some of the blurring effects of the earth’s atmosphere in the infrared, in visible light, there is still nothing that compares with Hubble in terms of the resolution that can be achieved in visible light. And there is also nothing in the near future that can compare with that.
So if you need this incredible high resolution, in pinpointing details and so on, things like Hubble is still the thing to [use]. Now, we will have the James Webb Space Telescope, which is a bigger mirror—Hubble is only 2.4 meters; James Webb is 6.5—it’s a much larger telescope. But it operates exclusively, essentially, in the infrared, not in visible light, like Hubble. So it will have its strengths. The LSSD will have its strengths, and so on. So I see this as really, a large effort, where observations from one observatory will complement the observations from another observatory. Because only through these different modes of observation—different wavelengths, different sensitivities and so on—will we be able to get a more complete picture.
What is dark energy?
We don’t know what this dark energy is. We know what it does: It accelerates the expansion of the universe. We also know that it’s a very smooth form of energy that fills the entire space. And we have some ideas as to what it might be. We think it’s the energy associated with empty space, with a physical vacuum. The vacuum, in physics, is not something where there is nothing there; it’s very, very active. In fact, it’s teeming with pairs of virtual particles and anti-particles that appear and disappear, appear and disappear, you know, and so on. So we think that it’s that energy of that vacuum that does this. The problem is that when we try to calculate how much energy there should be in the vacuum, we get numbers that are different by many orders of magnitude from what is actually seen. So we still don’t know exactly what this dark energy is, but the current best guess is that it is this energy of empty space, and that’s pushing the expansion of our universe to accelerate.
What will dark energy do to our universe?
Well, because we don’t yet know precisely how dark energy is going to behave, I cannot really answer that question. However, if dark energy is indeed the energy associated with empty space, then we actually know that its density stays constant, and that means that the expansion of our universe will continue to accelerate in the same way that it accelerates now. It will continue to accelerate forever. About a trillion years from now, or so, we would not be able—if there are astronomers still left in our neighborhood, I mean, of the galaxy—they would not be able to see any other galaxy in the night sky, even with their strongest telescopes. They would think they are the only galaxy in the universe about a trillion years from now. So the universe is going to go to a very cold death if that’s how things are.
Now, as I said, we still are not sure that dark energy is indeed this energy of empty space, so things can actually end up in very different ways. In one end, it can all end up in a big crunch, [where] the whole expansion turns back and starts re-collapsing. Or, it could end up in something we call, “a big rip,” which means that even the smallest structures will be torn apart [in] the end by the force of the expansion—even atoms and nuclei of atoms, you know, and everything and so on. But like I said, most observations to date are consistent with just continuing accelerating expansion, like now. You will not see any other galaxy and that’s going to be it.
What is dark matter?
Our universe is very strange. About 70 percent of it is in this form of energy we now call dark energy, which is this energy that is pushing the universe to accelerate. About 20-some percent is in the form of what we call dark matter, which is matter that we don’t see because it does not emit any light and it does not interact through the electromagnetic interaction. Less than 5 percent is what we call baryonic matter. That’s the stuff that we’re made of, [that] stars are made of, [that] galaxies are made of. That’s only less than 5 percent of the energy budget of the universe.
So dark matter is very important. It’s 20-some percent of the energy density of the universe. In particular, it has been instrumental in building up all the structures we see in the universe—galaxies and clusters of galaxies and so on. Now, because it interacts so weakly and does not emit any light, the question is how do you find that it’s there at all? And we find it through its gravitational interaction, because it does interact gravitationally. It started by looking at individual galaxies. You look at objects that are very far away from the center of the galaxy and if all the mass of the universe was the mass of the galaxy that we see, things there could not have stayed in orbit moving around the center of the galaxy; there just wasn’t enough mass to hold it there. So we infer that there must be all kinds of mass there that we don’t see. It started with that.
Then it went to clusters of galaxies, and we found that on all scales, there is this matter that we actually don’t see. Now, in some particular cases like in the case shown here, two clusters of galaxies collide. Each one of these clusters of galaxies has a halo of dark matter around it; but it also has some hot gas inside it—just normal gas, baryonic gas, inside it. Now, what happens when the two clusters collide is that the hot gas actually collides, it interacts electromagnetically and it collides. And because it’s hot, it can be seen in X-rays. So in this particular case, that is what is shown in red here and these are observations by the Chandra X-ray Observatory, in x-rays.
On the other hand, the dark matter—which interacts very, very weakly, does not interact thermally—it kind of passes through. And that’s the blue stuff we see here, which we don’t really see. We only know it’s there because what this matter does, [is] this thing we call gravitational lensing—namely, we look at objects that are far behind this cluster. The light from those objects is being distorted by the gravity of the cluster, in the same way that you put a lens in the path of light, it distorts the path of the light. This is one of the effects of Einstein’s general relativity.
So from this gravitational lensing, from the pattern that we see of the gravitational lensing, we can actually reconstruct the distribution of mass in these clusters of galaxies. And that’s what gives us this, where these blue things are. So those are the result of Hubble observations, of these distortions in the light of the more distant objects. And that tells us where the hot gas is. What you see here is essentially a clear separation between the two, because one just passes through, while the other actually collides. So with Hubble we have now been able, in a few cases, to map the distribution of dark matter in this way. And we’ve even been able to do a three-dimensional map, you know, across large distances in space of the distribution of dark matter.
What has Hubble discovered about black holes?
One of the things that Hubble discovered was that first of all at the center of almost every galaxy there is a black hole, a supermassive black hole, a large black hole. A discovery not by Hubble, by the way, is that at the center of our own Milky Way galaxy, there is a black hole with a mass of about 4 million times the mass of the sun. But Hubble showed that essentially, almost every galaxy you look at, there is a black hole at the center. But more than that, Hubble discovered that there is a very tight [relationship] between the mass of the black hole, at the center of the galaxy, and the dispersion of the velocities, of the speeds around that black hole—which is also a measure of the mass of the bulge of stars at the center of the galaxy around the black hole.
Now, this is very, very important because you might have thought that the galaxy and the black hole at its center don’t know of each other; they evolve completely independently. But the fact that the mass of the black hole is so tightly related to the velocity dispersion, or the mass of the bulge around it, means that the galaxies and the black holes actually co-evolve, they evolve together. And we think we understand now even how that works, that, you know, for as long as mass is being created there at the center, the bulge grows and the black hole grows. At a certain point you start to have this feedback where radiation starts pushing on the gas that is in there, and that stops the growth both of the black hole, if you like, and of the bulge. And so their masses remain tied together. This image here shows the galaxy M87, which has a black hole with a mass of about 3 billion times the mass of the sun at its center, and it has this spectacular jet also that we see comes out in this Hubble image from this.
Hubble has the ability to look very deep into the universe. What do Hubble deep fields show?
You take a very, very deep observation of a tiny area of sky—roughly the area that you would see if you looked through a drinking straw in the sky—and this is the Hubble Ultra Deep Field; it’s an image like this. Of course, the amazing thing in these images is that almost every point of light that you see here, with very few exceptions, is an entire galaxy with perhaps 100 billion stars like the sun. And there are many thousands of them in this image.
And we have gone deeper than the original Hubble Deep Field, especially because the Hubble Space Telescope now has capabilities also in infrared light. Infrared light allows us to see even deeper because the universe is expanding, so light is shifted more towards the red side of the spectrum. So if you look in the infrared you can see even deeper, you know, and so on. So we have gone deeper than this. We have now seen the universe as it was when it was even less than 500 million years old; when today it’s 13.8 billion years old. So we have really seen the universe at its infancy.
Now, what have we discovered from all of this? Well, first of all we have seen the whole history of how galaxies evolve, how they merge together. It’s all, you know, sort of mergers and acquisitions, basically, like big corporations. You start with small building blocks, they merge together to form bigger things, those merge together to form yet bigger things, until you form the large galaxies that we see today.
What does this ability to see so far into the universe mean for the discovery of other habitable planets?
Until 1992, we didn’t know of a single planet outside our solar system. In 1992, we discovered the first planets around another star, but they were around a bizarre star, a pulsar, which is this very compact object—not the type of thing where life could probably ever evolve, and so on. Only in 1995 did we discover the first planet around a more sun-like star. Since then, lots of planets have been discovered, in particular by the Kepler satellite.
There are now thousands of planet candidates and over 1,000 or so of confirmed extrasolar planets (planets that revolve around their parent stars). Not only that, Kepler has shown that statistically speaking, about maybe 20 percent of all stars, sun-like stars or smaller stars, have an Earth-sized planet, roughly, in the habitable zone around that star. The habitable zone is that zone which is neither too hot nor too cold around the central star, so that it can allow for liquid water on the surface, because we think liquid water may be an essential ingredient for life, so that’s why we call it the habitable zone. So about 20 percent of all the sun-like stars or smaller have something like an earth-sized planet in that habitable zone. That amounts to literally, billions of planets in the habitable zone in our own Milky Way galaxy alone.
Hubble has done something that is quite unique, and that is determine the composition of the atmospheres of some of these planets. How does it do that? The way it does that is for transiting planets—planets that happen to be along such a line of sight that the planet passes in front of its parent star. [These planets] eclipse its star. Now, that eclipse is very small because the planet is very small compared to the star. So all that it does is that the light of the star is dimmed by about 1 to 2 percent as the planet goes in front. However, and here is the trick, when the planet goes in front, yes, it dims the light of the star by about a couple of percent, but some of the light from the star passes through the atmosphere of the planet on its way to us. And then, we can look at what that atmosphere absorbs from the light of the star, and that tells us the composition of the atmosphere of this extrasolar planet.
This was done both with Hubble and with the Spitzer Space Telescope. We could determine what is the composition of the atmospheres of these planets. And we discovered now in many such, in particular so far, giant planets, like Jupiter in our solar system, we discovered water, we discovered even methane, some organics and so on. And a variety of other elements like sodium and so on were discovered in this thing. Now, I should say that in the upcoming years we will do even better because in 2017, there is this satellite TESS that is supposed to be launched, and that will discover a few such planets’ in habitable zones, and then in 2018, the James Webb Space Telescope, the scientific successor of Hubble, will be launched, and it will be able to characterize the atmospheres of those planets that TESS will find, those transiting planets. In principle, if we are really, really lucky and if life is ubiquitous, then we could perhaps even identify some biosignatures in those atmospheres. By that, I mean signatures that we think are most likely formed by life—for example oxygen, ozone, the atmosphere being outside of thermochemical equilibrium and things of that nature.
Now, mind you, even if we find a few biosignatures like water, like oxygen, ozone, and so on, this is not going to be, “Oh, we discovered life! That’s it!” because there are other processes that could in principle do all this. So any one biosignature is not going to be sufficient to convince anybody that we have discovered some life forms. But if we discover a whole combination of those, you know—we find an earth-sized planet, which is in the habitable zone, and we find that it has water, it has oxygen, it has ozone—in principle, we could even try to determine if there is something that chlorophyll does, you know, that plants do, there is some sort of rise, we call it the “red edge,” that you can see, in principle. It’s very hard to see, but in principle it can be seen. If we will find a whole combination of those and the atmosphere is out of thermochemical equilibrium, then maybe you can start to be somewhat convinced that there could be some life form there.
How has the prospect of completely decommissioning Hubble affected you?
Hubble has certainly been a large part of my scientific career; there is no question about that. Every servicing mission was extremely suspenseful and stressful for me. I once said that it’s a little bit like when your child is being born or something like that. I wasn’t exaggerating too much; I mean, I was exaggerating a bit, but I wasn’t exaggerating too much.
Now, I always knew that Hubble will finish working one day. In fact, nobody expected Hubble to work 25 years or more. By the way, Hubble is not done—we are celebrating 25 years now, but Hubble is doing fantastic science now, and in fact, has perhaps the largest complement of instruments it has ever had. So we definitely would like it to continue working at least I would say until 2020 or thereabouts, to allow it to overlap, maybe for a couple of years or so, with the James Webb space telescope. But I always knew that it will finish at one point, and it’s good to already have a scientific successor for Hubble, which is the James Webb Space Telescope.
The idea is really that science continues and you cannot get attached to a particular experiment so much [so] that when that experiment transforms into something even bigger and better, you would still lament the loss of the other one. So I think my transition to James Webb is gradual and a natural one, and I cannot wait to see that launched.