Ask any biologist—sex seems like a waste. It’s costly: Think of the enormous energy that goes into producing a peacock’s spectacular fan-shaped tail, apparently meant to entice a female to mate with him. And it seems inefficient: Sex allows us to pass on only half of our genes, and fully half the species (males) can’t bear children. Evolution is unsentimental, so those costs must bring benefits. The usual answer is that, by reshuffling genes with every generation, sex creates new genetic combinations, detaches beneficial mutations from harmful ones and gives a species a degree of evolutionary flexibility. It keeps genes in the pool that might not be of use today, but might save a creature’s descendants from plagues, pestilence, and parasites.
All that is probably true, but the thesis has one flaw. While the benefits of sexual reproduction tend to be subtle and become evident only over many generations, its costs are heavy and immediate. To understand sex completely, we need an explanation that goes back to the primordial soup of very early complex organisms and the immediate survival pressures they were under. Damian Dowling, an Australian evolutionary biologist, set forth a surprising idea last year with colleagues Justin Havird and Matthew Hall in the journal Bioessays. It proceeds from the simple fact that single-celled bacteria and archaea, known as prokaryotes, never indulge in sexual reproduction. They have some sex-like behaviors, including making bodily contact to exchange genes—sometimes called “bacterial sex.” But they do not reproduce sexually; they proliferate simply by dividing in two.
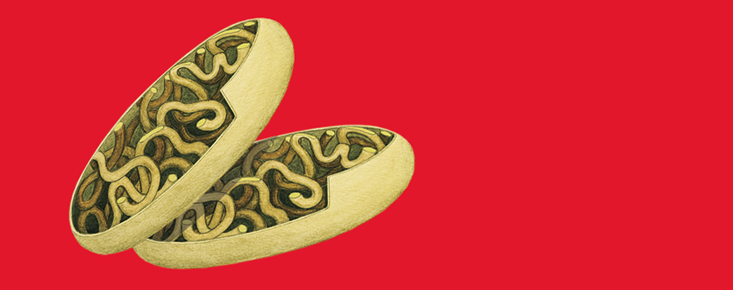
Sex is the privilege of more complex creatures, the eukaryotes. Organisms as diverse as amoebae and armadillos reproduce by dividing their chromosomes among gametes, such as sperm and ova, which in turn combine to create a new organism. The earliest well-preserved eukaryotes in the fossil record—red algae, dating back 1.2 billion years—are also the earliest known examples of sexual reproduction, as revealed by the presence of gametes. The defining characteristic of eukaryotes is that their cells are highly structured, containing not only a nucleus but also organelles—notably, mitochondria, those wondrous biological batteries that sequin our cells, providing us with the energy upon which our survival depends. “Our argument is simple: Eukaryotes are bound by two features—mitochondria and sex—and we believe there is a neglected link here,” says Dowling, who heads a team of researchers at Monash University in Melbourne, Australia.
While the benefits of sexual reproduction tend to be subtle, its costs are heavy and immediate.
That link lies in the fact that mitochondria are not just cellular batteries. Billions of years ago they were actually independent organisms. They are an example of how the human body is not entirely “human.” Our guts are full of trillions of foreign bacterial cells; our DNA is riddled with shards of old viruses; and even our cells are dollops of primordial soup. Scientists are increasingly realizing that many diseases are not external assaults, but imbalances of our inner ecosystem. In the case of mitochondria, conflicts can arise because these organelles contain their own unique and separate DNA. “Until recently, science has basically ignored the fact that we are all walking around with two genomes in every cell,” Dowling says, “that of our own nuclear genome, and that of the mitochondria.”
The mitochondrial genome tends to mutate quickly and is prone to fall out of sync with regulatory genes in the nucleus, with potentially negative consequences for the organism. Dowling thinks sex evolved as a way for the nucleus to keep pace with the protean realm it supervises. “The very empire that the early eukaryotes were building, based on their key weapon—the energy-producing mitochondria—was in jeopardy because mitochondria mutated so often,” he says. Sex constructs new genotypes with every generation and allows the nucleus to compensate when trouble arises. It is, in other words, a way to restore balance, to mend the divisions inside us. And unlike the other benefits of sex, this one was as important to the very first eukaryotes as to their descendants.
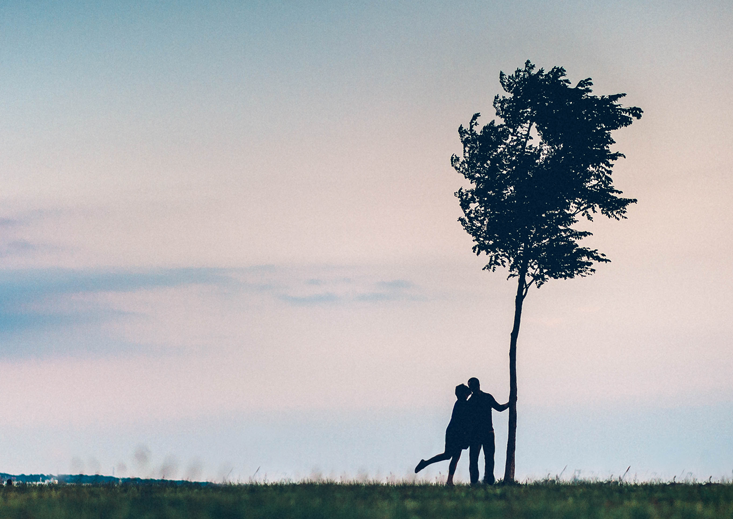
Around 2 billion years ago, two prokaryotes—two bacteria bobbing along in the primordial soup—engaged in what might be likened to the original sex act. One invaded the other. One ate, the other was eaten, and both lived to tell the tale. They merged, and over time, created something remarkable and new. The invader—the one that got eaten—evolved into the small but powerful mitochondria over the course of a few million years. The other likely evolved into the much larger nucleus.
This fusion resulted in a spectacular symbiosis. Mitochondria dedicated themselves to producing energy and were so efficient that an explosion of complex life on this planet soon fanned out in every direction. But specializing in power generation exacted a cost: Oxidative stress on a mitochondrion is high and can damage the organelle and its genes. Consequently, Dowling argues, mitochondrial DNA is “destined to accumulate mutations that are harmful.” Rapid mutation seems to be a generic problem of organelles that have maintained their own DNA, affecting not just mitochondria but sometimes chloroplasts (the photosynthetic organelles in plants, which themselves were once free-floating bacteria) as well. And recent research from Nils-Göran Larsson of the Max Planck Institute for Biology of Ageing in Cologne, Germany, suggests that mitochondrial replication is inherently error-prone (with the exception of specialized germ cells).
The high rate of mitochondrial mutation is seen in a wide variety of species today. In humans as in most other animals, mitochondria continually divide throughout our lifetime and, as they do, their genes mutate 10 to 100 times more rapidly than the genes in the nucleus. Not only does a single cell carry thousands of mitochondria, each mitochondrion contains multiple copies of its own DNA. The sheer number of changes is enormous.
Sex became the fastest way for a species to recover from a genomic mismatch.
To cope, over millions of years evolution moved most of the genes off of the mitochondria and into the far more stable nuclear genome. Today animal mitochondria have a mere 37 genes, all dedicated to producing energy. Most of their function is regulated and assisted by over 1,000 genes in the nuclear genome. But relinquishing genes provided only a partial solution. When those 37 genes falter or change, the cellular machinery can grind to a halt. Unless the nuclear genes that regulate them adapt, the cell can get sick and possibly die.
In 2007 Dowling and his colleagues studied what happens when the two sets of genes work at cross-purposes. In one experiment, they bred 23 generations of five different stocks of seed beetles, called Callosobruchus maculatus. In some strains, mitochondrial and nuclear genomes were adapted to work together. But when the experimenters transplanted mitochondria across strains, sperm viability declined. Dowling and his colleagues extended this study to fruit flies. They created five strains of fruit flies that differed only in their mitochondrial genes and studied the effects of these different strains on nuclear genes. Female flies were barely affected: only seven nuclear genes changed in activity. But the males had an astonishing 1,172 nuclear genes affected, mainly in the testes or sperm glands. “I almost fell over when I saw how strong the effect was on males,” says Dowling. “That’s about 10 percent of the male fly’s genome.”
The difference between the female and male responses has a natural explanation: Mitochondria are purely a maternal gift. Sperm do not pass them on; only a mother’s egg does. So, females with harmful mitochondrial mutations tend to die before they reproduce, sweeping those mutations out of the gene pool. But if such a mutation harms males without hurting females, it can persist. Dowling isn’t the only scientist who has demonstrated the profound interdependency of the mitonuclear duo. Clashing sets of genomes have also been shown to cause developmental delays and impaired fertility, in flies and sea crustaceans.
Humans, too, can suffer from jarring genomes. Evolutionary geneticist Dan Mishmar of Ben-Gurion University in Israel has found that mitonuclear conflict can increase vulnerability to Type II diabetes in Ashkenazi Jews who have certain genetic variations. A single mitochondrial mutation causes hereditary deafness in Arab-Israeli and Spanish families, according to neuroscientist Jan Willem Taanman of University College London. In some people who inherit the mutation, however, variants of nuclear genes are able to fix the problem, either by limiting the effect of the mitochondrial mutation or by compensating for it directly. Experiments with mice that are bred to have the same condition back this up: novel nuclear genes can restore hearing by bypassing the mitochondrial glitch, generating more energy to the ear’s cochlea.
Scientists now suspect that a hereditary form of progressive blindness (called Leber’s Hereditary Ocular Neuropathy) may be due in part to the dance between mitochondrial variations and the nuclear genome. Researchers have long been puzzled at the fact that this form of blindness doesn’t always show up with the same severity, or even at all, in everyone with the same mutation. For instance, a particular mutation in the mitochondrial DNA in Tibetans seems to protect against high-altitude stress and prevent this form of blindness, but at low altitudes can predispose people to it. How can this be? Could it be there is a different nuclear genome background? “We have a series of good candidates in the nuclear genome that might impinge on the mitochondrial DNA and explain this,” says neurogeneticist Valerio Carelli1 of the University of Bologna. Carelli has been studying LHON for 20 years. “The more completely we sequence genomes, the more granularity we reach to understand this issue,” he says.
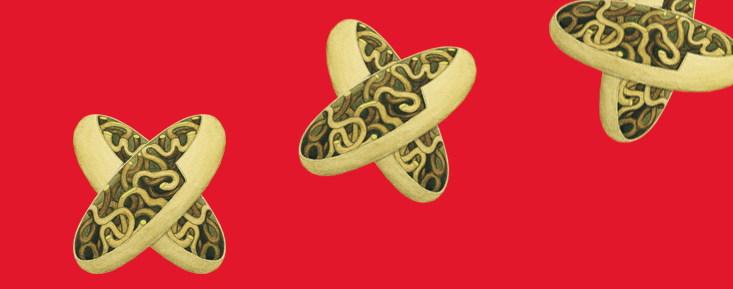
It was sex to the rescue. Sex became the fastest way for a species to recover from a genomic mismatch. Its genetic reshuffling creates novel variants that offer adaptations to all kinds of changes, both exterior and interior. “Sex is the only way that mitochondrial and nuclear genomes can stay in sync,” Havird says. “Without sex we’d have a situation where mitochondrial mutations accumulate much faster and the nucleus could not come up quickly enough with co-adapted mutations. Sex allows the eukaryote to get much more variation out of its genome because of the associated ‘trick’ of recombination.”
In addition to rejigging genes, sex provides a new mode of evolution. Unfit organisms are weeded out not just by environmental pressures, but also by the competition for mates, which is replicated on a microscopic level by the competition among sperm to fertilize an egg. This competition is a trial-by-fire for mitochondria and weeds out even the slightest mismatch. The midsection of a sperm cell is packed with mitochondria to fuel the winner-takes-all race up the vaginal canal. “Even though the mitochondrial genome is small, it’s centrally and fundamentally important to an individual’s biochemistry and fitness,” says biologist Matthew Gage, who is at the University of East Anglia in Norwich, England. “Mate choice and competition through sexual selection should improve mitonuclear matching in two ways. First, by selecting for high mitochondrial performance in males. Second, by selecting for high performance in their sperm, whose fertilizing ability is critically dependent on optimal mitochondrial function.”
As a test of his theory, Dowling looks across species. The mutation rate of mitochondria varies hugely from algae to tulips to coral. Dowling’s theory predicts that the faster the mitochondrial mutation rate is, the more often the members of that species will need to have sex. Dowling thinks the evidence supports his view. Almost all animals have high mitochondrial mutation rates and require sex to reproduce, whereas plants are mellower on both counts. “Many land plants have exceptionally low mitochondrial mutation rates, and in fact, very few plants require sex to reproduce,” Dowling says. “Almost all of them can have sex when they need to, but they can also asexually reproduce.
Others aren’t so sure. Evolutionary biologist Bram Kuijper of University College in London is a fan of Dowling’s new theory, but wants to see better proof. “We know so little about mitochondrial mutation rates over a whole range of organisms,” he says. Biologist David Roy Smith of the University of Western Ontario agrees. “Although in general the mitochondrial genomes of animals mutate more quickly than those of plants, it gets complicated very quickly. Recent studies have shown that the mutation rate across a single plant mitochondrial genome can vary by nearly three orders of magnitude.” One species Kuijper would like to see tested is microsporidia. These tiny parasites are eukaryotes, but lost their mitochondria at some point in their evolutionary past. “Do they have sex?” he wonders. “If so, how much?” And on the opposite end of the spectrum are bdelloid rotifers, small aquatic bugs that have mitochondria, but may not reproduce sexually. University of British Columbia theoretical biologist Sarah Otto, whose mathematical models of evolution have won her a MacArthur Foundation award, worries that these bugs are the exception that disproves the theory. “There’s a lot of evidence they still engage in asexual gene transfer,” she says. But she says Dowling may be right that sexual reproduction evolved in response to the symbiotic relationships among the creatures that merged to form eukaryotes. Otto says, encased in extra membranes, “gene exchange became rarer, so rare that the advantages of evolving a sexual system became strong enough to counter its costs.”
This highlights a broader point about evolution: Partnerships among organisms create the impetus to change. “The great biologist Lynn Margulis taught us that most of the big jumps in evolution come about by symbiosis,” says Freeman Dyson, who, though best known as a physicist, ranges into many subjects and authored the small masterpiece, Origins of Life. A host creature is invaded by a parasite, explains Dyson. The two then struggle, in a fight nearly to the death, that unexpectedly results in new, astonishing life. “Since the host is providing life-support to the symbiont,” Dyson explains, “the symbiont is free to evolve, gaining or losing genetic capabilities randomly and rapidly. Rarely, the symbiont invents new structures that dramatically change the lifestyle of the host.”
When it comes to a dramatically changed lifestyle, sex certainly fits the bill. With its pleasures and plumage, its elaborate and often exhausting mating rituals, it has the power to smash up and patch up lives. All of that turmoil might, in its own way, be an effort to avoid the deeper turmoil caused by a thimbleful of 37 genes.
Jill Neimark is an Atlanta-based writer whose work has been featured in Discover, Science, NPR, Nautilus, Aeon, Quartz, Psychology Today and The New York Times.
The lead photocollage was created from an image from De Agostini Picture Library / Getty Images