This summer, NASA’s Perseverance rover will set out on a voyage to the edge of the Jezero crater on Mars. The goal of the mission is to learn more about our neighboring planet, and to collect core samples that will one day return to Earth. The hope is that by studying the ancient carbonate rocks that line the northern edge of the crater, we might glean signs of life. Anything we find there, even fossilized remnants of a more verdant past, may offer priceless clues about the initial spark of life on our own planet.
Mars is big, and each costly mission can only cover so much ground—so some guiding principles are necessary to narrow the search. Even if we assume life on Earth and Mars arose independently, we can look to the most ancient forms of life on Earth for clues about where and what to look for on the red planet. So far, the favored approach has been to focus on a common behavior of all known life forms: the ability to harvest energy from the environment. What has emerged from the study of diverse metabolic systems is that the myriad tactics by which cells accomplish this feat all boil down to a shared strategy. In a word: electricity.
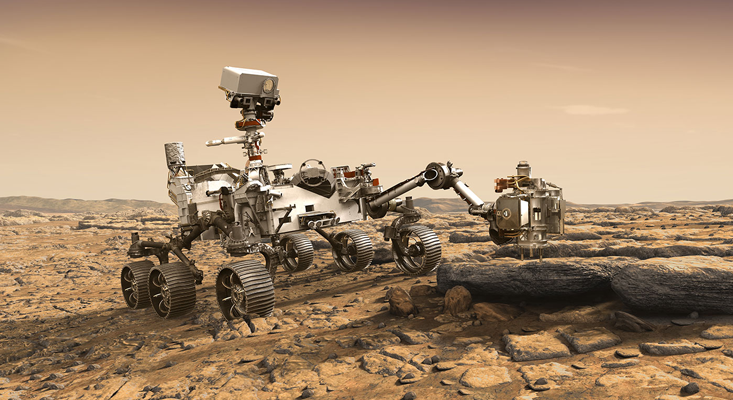
Electricity is commonly considered a human technology: a carefully crafted network of circuitry woven throughout civilization, designed to power our needs. But think of a thunderstorm arcing a bolt of lightning across the sky, a crystal of pyrite slowly rusting, an oilfield burning—humans didn’t invent electricity, no matter how much we’d like to think so. It precedes us, and life itself, as an inanimate physical process. It is also at the core of how organisms access the motivating force for life—energy.
Energy is a measure of the ability to do work, and cells, the fundamental unit of life as we know it, have much work to do. They construct proteins, copy themselves, and move against the ubiquitous pull of gravity. In the biosphere we’re familiar with, organisms depend upon the sun to bankroll their work—either directly through photosynthesis, or by consuming its organic products. Both of these processes are fundamentally electrical. So is the metabolism of the deep biosphere, a parallel world beneath our feet1 that thrives in the dark. Thousands of meters down, scientists have found bacteria that electrify themselves by eating and breathing simple geofuels—a clue, perhaps, for where to begin the search for life on a planet like Mars.
It’s hard to rule anything out when you’re working on the scale of the universe.
The surface of Mars is almost universally considered untenable for living organisms. But beneath the surface, where there is liquid water, warmed by remnant geothermal activity and radiation emanating from a slowly cooling core, scientists suspect they might find conditions similar to those of our deep biosphere. And if cells on Earth can harness electricity under such conditions, perhaps so can cells on Mars.
On Earth’s surface, many organisms produce electricity by shuttling charge from glucose to oxygen. Underground, they can use hydrogen and carbon dioxide. But the operation that generates electricity in both cases is the same—an equilibration of charge between two compatible compounds. After all, electricity is nothing more than energy derived from either static or dynamic charge. But what exactly is charge, and how does life use electricity to do work?
“Positive” and “negative” charges reflect observable physical properties of the atoms involved in electricity, just like “hot” and “cold” indicate molecular temperature. Imagine two reservoirs, one “hot,” or “negative,” and one “cold,” or “positive.” When they’re separated, no work can be done. But bring them into contact, and the motion that arises in the bridge between them can be harnessed to do work. The same is true for the negative and positive terminals of an electric circuit. The difference in charge between the two terminals is known as voltage, and the flow of current between them can be used productively. On Earth, more ancient, deep biosphere bacteria utilize low voltage circuits, while the more complex surface-dwelling organisms depend upon higher voltages. Therefore, when it comes to sniffing out life on Mars, we should expect to find simpler, less-evolved, low-voltage microbes away from the surface.
Even such an elementary microbe, which harvests electricity from geological gases percolating through the Martian crust, is likely to possess a conserved metabolic circuit—given all known Terran organisms use the same mechanism to power themselves. All cells that have been studied bridge the charge difference between what is eaten and what is breathed with a biological wire known as the electron transport chain (ETC). The universality of the ETC suggests it was an early innovation in the evolution of life on Earth, and suggests that it’s the best solution to the problem at hand. Assuming the origin of life on Mars mirrors our own, we can expect to find some version of the ETC, and any differences will tell the story of two evolutionary paths.
If the systems are fundamentally similar, either on the basis of nucleic or amino acids, it might indicate that Martian life is of terrestrial origin, brought to the red planet during the period of Heavy Bombardment, a time of increased asteroid impacts thought to have happened approximately 3.8 billion years ago. But there’s another alternative, and that’s the mechanism at the center of all living cells evolved this way because it’s the only way it could have happened.
Although it seems like evolutionary determinism, there’s some weight to the idea that cells are constrained to a certain evolutionary path, no matter the environment they appear in. Certainly, the charge-transfer reactions central to metabolism on Earth, more broadly known as reduction/oxidation (redox) reactions, are known to produce electric current even in the absence of biology. Take for example the galvanic cell, invented in the 1700s by Alessandro Volta. The redox reactions central to his invention remain vital to the ubiquitous modern-day battery. What biologists have found since his discovery is that similar redox reactions are at the center of metabolism. Except instead of the charge being derived from metals, nature provides a wide range of different substances that can be eaten and breathed.
Thousands of meters down, bacteria electrify themselves by eating simple geofuels.
All kinds of compounds, everything from hydrogen gas to sulfate—can serve as the terminals of a metabolic circuit. Despite this flexibility, the depth of structural and functional similarity in the ETC across a multitude of life forms suggests only a few degrees of freedom during the system’s evolution. Annette Rowe is head of the Electromicrobiology Laboratory at the University of Cincinnati, where she studies the often unusual ways that organisms power their metabolic circuits. Some of her research has focused on bacteria capable of breathing current carried by electrodes.2 Reached by phone, Rowe points out that while the metabolic systems of two organisms may “have protein architecture that looks really similar, most of them are uniquely derived, evolutionarily speaking.” This means that the same solution to the problem of distributing the electric harvest throughout the cell has appeared over and over again throughout history. The name of that solution? Adenosine triphosphate.
Adenosine triphosphate, ATP for short, is one of those incredible pieces of biology that appears to be universal. There is no exception to the rule that to live is to work, and no exception to the rule that all known cells use electrochemical gradients to do work. But most internal cellular processes do not access electricity directly. Instead, they channel the electric power into a mobile intermediary—ATP—for much the same reason that we prefer wireless technology. Dragging a cord about is prohibitively restrictive. Internal cellular processes like active transport, polymerization, and locomotion occur far from metabolic machinery. Instead of depending on a tangle of wires, cells use diffusible ATP to provide the necessary kick. “ATP is a fundamental currency to life as we know it,” Rowe says. In much the same way that human currency can be universally exchanged for services in society, ATP can easily be exchanged for work within the cell.
ATP is a high-energy, spring-loaded molecule that wants, more than anything else, to burst apart. This explosive ability is used by proteins to perform mechanical processes. ATP, like the electron transport chain, appears to have evolved many times over. The robust nature of this evolutionary convergence suggests that we would find ATP, or a similar intermediary, in extraterrestrial life.
Living cells evolved this way because it’s the only way it could have happened.
The question that remains is whether the patterns we observe on Earth—the electron transport chain and its role in the production of ATP—are fundamental to all of life, or just life as we know it. Finding alternative systems evolving on Earth in real-time is difficult because protocells,3 precursors to the simplest microbes, would be outcompeted by even the slowest growing contender, backed by 3.8 billion years of evolution. It might be that forerunners to life are abundant in our deep biosphere, but predation prevents them from developing. Exploring the low-energy depths on Mars, where potential biology moves slower, might uncover ancient structures that fill in the gaps in our understanding of the earliest forms of life on both planets.
Searching below the surface of Mars to shed light on the emergence of life is not exactly a new idea. Thomas Gold, a 20th-century polymath who predicted the existence of the deep biosphere almost a decade before it was discovered, suggested that “deep, chemically supplied life … may be very common in the universe.”4 Recent models, indeed suggest the presence of some 6 billion Earth-like planets in our galaxy alone,5 which hints that the low-voltage chemistry thought to have supported early life on our planet may be widespread throughout the cosmos. Gold’s principle may readily apply to the subsurface regions of several bodies within our own solar system, including Mars.
Another enticing proposal by Gold is that our definition of life might be limited by our experiences. He suggests that underneath the known deep biosphere “there will generally lie a large domain that is too hot for the bacterial life we know, but that is nevertheless capable of supporting other systematic chemical processing systems that can mediate those energy reactions.” In other words, there could be other paradigms that stretch our very understanding of life itself.
Other scientists are on the same track. Although life as we know it is powered by electricity, it’s conceivable that any energetic gradient might fuel the spark. Rowe cautiously speculates that “life as we can imagine it happens to use redox reactions, and so people look for [them] when searching for life on other planets. But there may be other ways to get energy—thermal or magnetic processes—ones that don’t seem viable for life at the outset, but who knows? It’s hard to rule anything out when you’re working on the scale of the universe.”
Still, it seems most researchers have their bets on something more familiar to us back home. Reached by phone, David Flannery, a research fellow at Queensland University of Technology, working on the Perseverance NASA mission, says, “We know now the deep biosphere is extensive. So it seems reasonable to assume Mars would have had a similar biosphere if life ever existed there in the past.” On Earth, there is a good chance that biology evolved outward from the depths. It will take us, or one of our rovers, drilling deep into the Martian crust, to reveal the potential roots of a second tree of life.
Michael Shilo DeLay completed his graduate work at Columbia University investigating the mechanics of nano-confined water. Anastasia Bendebury holds a Ph.D in bacterial multicellularity from the same university. Together, the authors run Demystifying Science. You can follow them on Twitter @Demystifysci.
References
1. Deep Carbon Observatory: A Decade of Discovery. Deepcarbon.net (2019).
2. Nealson, K.H. & Rowe, A.R. Electromicrobiology: Realities, grand challenges, goals and predictions. Microbial Biotechnology 9, 595-600 (2016).
3. Jordan, S.F., et. al Promotion of protocell self-assembly from mixed amphiphiles at the origin of life. Nature Ecology & Evolution 3, 1705-1714 (2019).
4. Gold, T. The deep, hot biosphere. Proceedings of the National Academy of Sciences 89, 6045-6049 (1992).
5. Kunimoto, M. & Matthews, J.M. Searching the entirety of Kepler data. II. Occurrence rate estimates for FGK stars. The Astronomical Journal 159, 248 (2020).
Lead image: Tiko Aramyan / Shutterstock