The only intelligent life forms we know of reside here on Earth. But that hasn’t stopped us from trying to answer the question: “Are we alone?”
The search for extraterrestrial intelligence now has an accepted acronym, SETI, and a growing number of interested participants. The odds of galactic company can only be estimated, and a primary tool to predict the number of other civilizations out there is the Drake Equation. Nearly everyone interested in SETI has heard of it. But views of its utility vary widely. To some, it is a useful way to estimate the number of technological civilizations in our galaxy and the chances of detecting an extraterrestrial message. Others view it as a wasted effort, given the huge range of conjectures involved in its components. There is a middle ground to use it to update and assess the reliability of relevant data that we have and the ways to improve upon the uncertainties. It can certainly be an effective tool for stimulating curiosity on this subject.
Frank Drake presented the equation in 1961 at what may have been the first formal SETI conference at the National Radio Astronomy Observatory in West Virginia. The intent was likely to stimulate discussion and evaluate proposed research, not to arrive at a true estimate of the number of intelligent ETs whose signals we could detect by multiplying its components. In its original form, the Drake Equation is:
N = R* x fp x ne x fl x fi x fc x L where:
· N is the number of intelligent civilizations in the Milky Way Galaxy, who, for this exercise, emit radio, light, or other transmissions that are detectable from afar.
· R* is the rate of star formation per year for the galaxy.
· fp is the fraction of those stars with planets.
· ne is the average number of planets capable of supporting life (think of e for “earth-like” or “ecologically fit”).
· fl is the number of those that actually develop life.
· fi the number of those where life becomes intelligent.
· fc the fraction of those emitting detectable signals into space
· L the lifetime of a communicating civilization.
Multiplying the terms would yield estimates that range from a galaxy teeming with ETIs (extraterrestrial intelligent beings) to us alone. Though ET has yet to phone in, advances in astronomy since 1961 have firmed up the estimates of some of these terms. Let’s revisit each of them.
N is usually considered the number of communicating civilizations in just our Milky Way galaxy. But keep in mind that there are hundreds of billions of other galaxies and their stars much farther away in the visible universe.
The factor R* is estimated ~1 by some astronomers, based on the current rate of star formation in our entire galaxy. But the rate of star formation was once higher, and dividing the number of stars in the galaxy (200-400 billion) by its estimated age (roughly 10 billion years) would yield an R* closer to 10 per year, the number I will choose to plug in.
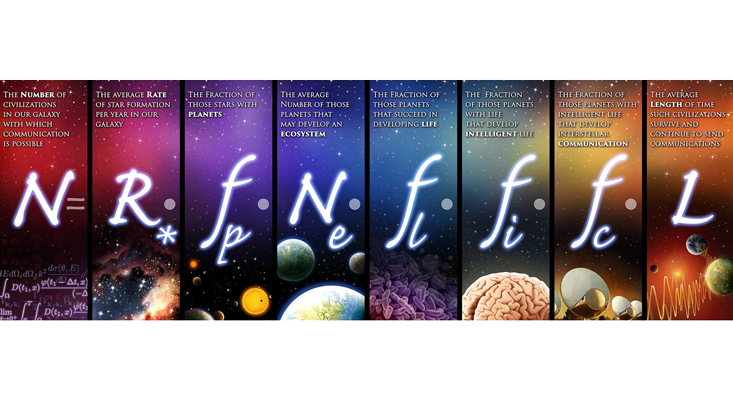
fp is one of the more constrained fractions because of recent advances in astronomy. Even a decade ago it was a conjecture, based on the theoretical likelihood that gas and dust left over from the birth of new stars would coalesce into planets. But data is now available. We have detected planets orbiting stars by observing the wobble in the Doppler shift of their spectrum as orbiting planets tug their sun to and fro during their orbit. We can also directly observe stellar eclipses as the planet passes in front of its home star. This produces a periodic dimming of the star light, even though we don’t have telescopes powerful enough to actually see the dark round disc of the planet against the background star. Following ground-based observations, the recently completed Kepler Satellite mission has scored thousands of extra-solar planets.
With this data, astronomers now claim that roughly half of stars have planetary systems, each of which is likely to contain more than one planet. The current champion is the TRAPPIST-1 system, located a mere 39 light-years from Earth, with 7 rocky planets in orbit. Keep in mind the limitations of current searches and the selection effects involved. Only two types have stars (relatively low mass M and G class stars) have been more thoroughly searched, so extrapolations to other star types are required. In addition, it’s the large planets and ones orbiting close to their sun that are easiest to spot, since they will cause more gravitational wobble on their star and be more likely to cast a detectable shadow as they cross in front of it. Therefore, many small, “Earth-like” planets have escaped detection. It’s probably safe to plug in the estimate of fp = 0.5, though most astronomers might now conclude that the total number of planets actually exceeds the number of stars out there.
Estimates of ecologically fit for life, ne, are often said to mean that the planet orbits in the “Goldilocks zone” where it has a surface temperature just right to have liquid water. This is a reasonable prerequisite for life, though alternate scenarios are possible.
For example, spacecraft have recently found that Saturn’s moon Enceladas and Jupiter’s moon Europa have liquid oceans beneath icy crusts which might conceivably harbor life, though not likely to be the kind that would broadcast radio or lasar signals into the cosmos. Requirements in addition to having the right distance from a star of given luminosity would reduce ne. This list of possible prerequisites for an Earth-like ecology includes: having the right kind of atmosphere to stabilize the surface temperature (avoiding a runaway greenhouse effect that has super-heated Venus), having an atmosphere that is “breathable” for its life-forms, having both open oceans and solid land, possessing a large moon to stabilize the planet’s rotation, and a few more. I allow these fitness extras to reduce my estimate of ne from nearly 1 to about 0.5.
Personally, I like the odds that planetary life begets intelligent species and that they communicate into space.
Now we get to speculate about life. Many scientists claim that life formed “soon after” the Earth solidified, and its surface cooled from an original molten state resulting from its birth pangs of rocky collisions and supercharged volcanism roughly 4 billion years ago. “Soon” in this case means a few hundred million years. If so, the advent of life might be thought of as “easy” and fl is near 1. But there is more to this issue. If one seeks an evidence based-value of fl, the geological proof of the earliest life is uncertain. Rocks that are reliably dated by radioactive decay still don’t contain the kinds of recognizable fossils that we come to expect from far later life forms. It has been argued that regular microscopic features in rocks more than 3.8 billion years old were formed by bacteria-like organisms in that era, but non-living mineral processes could also account for them, and the debate is on. More generally accepted evidence of life does appear in the geological record about 3.5 billion years ago.
Another way to estimate fl considers the chemistry and biology of the origin of life. This process is far from solved. Landmark experiments have shown in the lab that organic molecules, including amino acids and nucleic acids (the eventual building blocks of proteins and RNA and DNA), can form from simple precursors like carbon dioxide, ammonia, water, and other inorganic molecules. Such building blocks of life have now been observed in interstellar clouds, meteorites, and comets. However, to create the first living cells required a confluence of molecules fighting entropy to extract energy from sunlight (or alternative sources of heat) to sustain their molecular engines and build molecular structures. Plus, at some point, these linked chemical reactions and “proto-cells” had to find a way to reproduce and encode the instructions for building future generations. This seems like a hard task. But given time, space, and many opportunities to try, we know it has happened at least once. Thus, some contend that life will form wherever it can and fl = 1, while others argue that it is very unlikely and represents a bottleneck in the Drake Equation.
Choosing odds from an example of one is a hazardous exercise. Indeed, this conundrum supports the scientific value of searching for even primitive life forms beneath the surface of Mars or in the oceans that underlie the ice layers on the moons of Jupiter and Saturn. If we found that life arose independently on two or three different planets or moons in our solar system, I would revise my guestimate of fl to 1. But for this exercise, I will choose a conservative value of fI = 0.1, rather lower than the current popular choice.
Personally, I like the odds of the next two components in the equation, fi and fc, that planetary life begets intelligent species, and that they eventually communicate into space. Once life has gotten going, Darwinian evolution seems likely to set in. It’s called natural selection because it really is. The best functioning varieties that produce the most viable descendants win out in the game of life. Of course, that’s an over-simplification of modern evolutionary theory, but the kernel of truth is there. Evolutionists use the term “adaptive” to refer to a trait that offers a Darwinian advantage in surviving numbers of the like and in the ability to morph into new species. Evolution is unpredictable and choosing a value of fl approaches guesswork. (One sobering thought is that life on Earth remained microbe-only for nearly 3 billion years before multicellular animals arose.) I think, however, that intelligence is powerfully adaptive. So, I am willing to be optimistic and guess 0.5 for fi. Perhaps this is an over-estimate, given the possibility of planet-scale catastrophes such as the asteroid collision that killed off the dinosaurs and many other species 65 million years ago, which was only one of several mass-extinction events that we know of in Earth’s history.
Next, what are the odds that intelligent species communicate with other planets? It’s not fc = 1. Dolphins are certainly intelligent, but they are unlikely to build powerful radio transmitters and point them skyward. I set fc = 0.5, hoping that half the planets that have evolved intelligent species sport at least one life-form that signals outward either intentionally or inadvertently. This is guesswork, and it is risky to presume human-like attitudes and abilities onto alien species.
So far, my personal tally for all of the Drake factors is 0.0625.
We now come to L. By our definition of a technological (transmitting) civilization, we have been around for about 100 years. How sanguine are you about the future of Homo sapiens? Or for that matter, do you foresee the evolution of a more stable intelligent life form on Earth? Part-cyborg/part human? Or just a triumphant computer with self-repairing capability? And are you more pessimistic and presume that a technological civilization becomes inherently unstable once it invents weapons of mass destruction and the means to ruin the ecology of its home planet? Do you favor L = 500 years, or 5,000, or 5 million, or 5 billion? Do you bump this up by allowing repeated episodes of development of technological civilizations on a planet following an Armageddon? The range of uncertainty of L swamps that of all the other factors in the Drake Equation. This is perhaps the greatest value of the exercise. I personally can’t come up with a more precise conjecture than between 500 and 5 million years.
The bell-shaped curve of probability indicates ET will be millions to billions of years farther along in its trajectory of evolution than us.
So, with all of the above considered, my current guess for N ranges from about 30 to 300,000. In other words, our closest neighbors might be well across the galaxy from us or amongst the nearest stars. That’s not very predictive and awfully dependent on one’s mood and the news of the day.
For further consideration, a number of extensions have been suggested to revise the original Drake Equation. Might not even a rare super-advanced civilization spread throughout the galaxy and show up in many places (perhaps humanoid, with slightly different masks on their faces, a la Star Trek)? On the other hand, what if ET does not want to bother to communicate to other planets? And if it does, would we be able to comprehend anything a vastly advanced intelligence would try to say to us? Yes, we heard from someone, but the message seems like pure gibberish, or resembles how your cat perceives your learned conversations.
This could be a serious issue. Will we know for sure we are hearing a signal from ETIs rather than “noise” from natural astronomical objects or from local chatter? And will we be able to understand a message? The odds dictate that the ETs we hear from will be extremely advanced compared to us. We have been capable of interstellar signaling for about 100 years, counting from the first radio and TV shows through the advent of radio telescopes and powerful laser bursts. Stars like the sun last for about 10 billion years (some far longer and some far shorter, which might have insufficient time to evolve technological life). So, the bell-shaped curve of probability indicates that it is far more likely that we receive a message from an ET who will be millions to billions of years farther along in its trajectory of evolution than us. The odds of hearing from someone a more comfortable few hundred years ahead of us are miniscule, and those more primitive than us are unable to send radio signals. Will we understand these hyper-advanced beings? Will they bother to make themselves understood to primitive Earthlings? (I presume that the burden of having comprehensible conversation should fall on the more advanced creatures. In the otherwise wonderful science-fiction movie Arrival, I was puzzled that it was left up to Amy Adams to figure out the language of the visiting cloud creatures, rather than the other way around.)
The Drake Equation was formulated to estimate the number of ETI civilizations out there. Just learning that we are not alone will be mind-blowing enough, but why not tack on the odds that we will understand a message from them? And what about the odds that while someone decodes a message, much of humanity will ignore it or not believe it? After all, there is still a Flat Earth Society. The science-fiction writer Arthur C. Clark famously said that any sufficiently advanced technology will be indistinguishable from magic. Others have extended this axiom to state that any sufficiently advanced extraterrestrial will be indistinguishable from God.
In the end, the Drake Equation is not a vehicle for precise predictions and budget planning. It’s been said it was never meant to be solved. It’s value lies in the thought-provoking question marks. After all, no one is asking Congress to devote a sizable percentage of the national budget to SETI, nor even a large percentage of the NASA budget. But it shouldn’t be zero percent, as it is now. The pay-off is much too great to devote zero effort to the search. And we certainly have not exhausted the search. If N = 100,000, it means we should search more than 3 million stars at multiple wave lengths to have a solid chance of making contact. To paraphrase Carl Sagan, our chances clearly improve if we actively seek out a signal, rather than merely “waiting for the flying saucer to land in Harvard Square.”
Richard Lawn is a former astronomy student at Harvard who received a Ph.D. in molecular biology. His career in biomedical research included some of the first isolation and characterization of human genes which contributed to both basic research goals and to the development of treatments for a number of inherited diseases including hemophelia and anemia, while at CalTech, Genentech, Inc., Stanford and CV Therapeutics, Inc. He has recently returned to his first scientific love of astronomy and is working with the SETI group at the University of California, Berkeley. He writes regular articles on hot topics in astronomy and the search for extraterrestrial life on SETI@home, where an earlier version of this essay appeared.
Lead image: Design Projects / Shutterstock